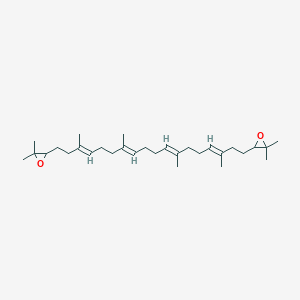
Dioxidosqualene
描述
Dioxidosqualene is a naturally occurring compound found in plants, fungi, and animals. It is a precursor to sterols and other lipids, and is essential for the production of many important molecules in the body. Dioxidosqualene is a key component in the synthesis of cholesterol and other steroid hormones, as well as the formation of vitamin D and other biological molecules. In addition, it is important for the production of carotenoids, which are essential for photosynthesis in plants. Dioxidosqualene is also found in some foods, such as olive oil, and is used in the production of cosmetics and pharmaceuticals.
科学研究应用
Role in Triterpene Biosynthesis
Dioxidosqualene plays a crucial role in the biosynthesis of triterpenes . It is a substrate for the enzyme Oxidosqualene Cyclase (OSC), which catalyzes the cyclization of 2,3-oxidosqualene into sterols and triterpenes . This process is the first committed step of triterpenoid biosynthesis .
Defense Against Biotic Stressors
Triterpenes, which are synthesized from dioxidosqualene, play pivotal roles in plant defense against biotic stressors . They are part of the plant’s “weapons” to conquer various challenges from the surrounding environment .
Pharmacological Applications
Triterpenes, derived from dioxidosqualene, have multiple biological activities, including functional foods and drugs . They are a class of bioactive compounds with diverse biological functions .
Role in Plant Evolution
The long evolutionary process of plants has been associated with the development of secondary metabolites like triterpenes . The biosynthesis of these compounds, which involves dioxidosqualene, has helped plants cope with various challenges.
Influence on Triterpene Glycoside Compounds
The initial steps of triterpene biosynthesis, which involve dioxidosqualene, influence defense against certain pests through the modulation of downstream triterpene glycoside compounds .
Potential Role in Saponin Biosynthesis
Dioxidosqualene is potentially involved in triterpenoid saponin biosynthesis . Saponins are a class of chemical compounds found in particular abundance in various plant species. They have properties that make them suitable for various applications, including medicinal uses.
作用机制
Target of Action
The primary target of Dioxidosqualene is the enzyme Oxidosqualene Cyclase (OSC) . OSCs are key rate-limiting enzymes in the biosynthesis of triterpenes . They catalyze the cyclization of 2,3-oxidosqualene into sterols and triterpenes with different skeletons .
Mode of Action
Dioxidosqualene interacts with its target, OSC, by serving as a substrate for the enzyme . In the enzymatic mechanism, the 2,3-oxidosqualene substrate adopts a chair–chair–chair conformation, leading to the formation of the dammarenyl carbocation intermediate before cyclization . This gives rise to various triterpenoid types such as ursane, oleanane, lupane, and other diverse skeletal structures .
Biochemical Pathways
Dioxidosqualene plays a crucial role in the triterpene biosynthetic pathway . It is involved in the initial steps of triterpene biosynthesis, subsequently influencing defense against certain biotic stressors through the modulation of downstream triterpene glycoside compounds . The cyclization of 2,3-oxidosqualene is a pivotal step in triterpenoid biosynthesis, catalyzed by OSC enzymes in plants .
Pharmacokinetics
Computational methods can significantly advance the study of chemical admet properties, offering both an economical and a time-efficient approach .
Result of Action
The molecular and cellular effects of Dioxidosqualene’s action involve the production of various triterpenoids . For instance, in cancer cell lines, the inhibition of SQLE, a target of Dioxidosqualene, caused a toxic accumulation of the substrate squalene . Moreover, certain triterpenes within clusters displayed a significant negative correlation with larval mass, indicating potential toxic effects .
属性
IUPAC Name |
3-[(3E,7E,11E,15E)-18-(3,3-dimethyloxiran-2-yl)-3,7,12,16-tetramethyloctadeca-3,7,11,15-tetraenyl]-2,2-dimethyloxirane | |
---|---|---|
Source | PubChem | |
URL | https://pubchem.ncbi.nlm.nih.gov | |
Description | Data deposited in or computed by PubChem | |
InChI |
InChI=1S/C30H50O2/c1-23(15-11-17-25(3)19-21-27-29(5,6)31-27)13-9-10-14-24(2)16-12-18-26(4)20-22-28-30(7,8)32-28/h13-14,17-18,27-28H,9-12,15-16,19-22H2,1-8H3/b23-13+,24-14+,25-17+,26-18+ | |
Source | PubChem | |
URL | https://pubchem.ncbi.nlm.nih.gov | |
Description | Data deposited in or computed by PubChem | |
InChI Key |
KABSNIWLJXCBGG-TXLDAEQNSA-N | |
Source | PubChem | |
URL | https://pubchem.ncbi.nlm.nih.gov | |
Description | Data deposited in or computed by PubChem | |
Canonical SMILES |
CC(=CCCC=C(C)CCC=C(C)CCC1C(O1)(C)C)CCC=C(C)CCC2C(O2)(C)C | |
Source | PubChem | |
URL | https://pubchem.ncbi.nlm.nih.gov | |
Description | Data deposited in or computed by PubChem | |
Isomeric SMILES |
C/C(=C\CC/C=C(/CC/C=C(/CCC1OC1(C)C)\C)\C)/CC/C=C(/CCC2OC2(C)C)\C | |
Source | PubChem | |
URL | https://pubchem.ncbi.nlm.nih.gov | |
Description | Data deposited in or computed by PubChem | |
Molecular Formula |
C30H50O2 | |
Source | PubChem | |
URL | https://pubchem.ncbi.nlm.nih.gov | |
Description | Data deposited in or computed by PubChem | |
Molecular Weight |
442.7 g/mol | |
Source | PubChem | |
URL | https://pubchem.ncbi.nlm.nih.gov | |
Description | Data deposited in or computed by PubChem | |
Product Name |
3-[(3E,7E,11E,15E)-18-(3,3-dimethyloxiran-2-yl)-3,7,12,16-tetramethyloctadeca-3,7,11,15-tetraenyl]-2,2-dimethyloxirane | |
CAS RN |
31063-19-1 | |
Record name | 2,3,22,23-Dioxidosqualene | |
Source | ChemIDplus | |
URL | https://pubchem.ncbi.nlm.nih.gov/substance/?source=chemidplus&sourceid=0031063191 | |
Description | ChemIDplus is a free, web search system that provides access to the structure and nomenclature authority files used for the identification of chemical substances cited in National Library of Medicine (NLM) databases, including the TOXNET system. | |
Retrosynthesis Analysis
AI-Powered Synthesis Planning: Our tool employs the Template_relevance Pistachio, Template_relevance Bkms_metabolic, Template_relevance Pistachio_ringbreaker, Template_relevance Reaxys, Template_relevance Reaxys_biocatalysis model, leveraging a vast database of chemical reactions to predict feasible synthetic routes.
One-Step Synthesis Focus: Specifically designed for one-step synthesis, it provides concise and direct routes for your target compounds, streamlining the synthesis process.
Accurate Predictions: Utilizing the extensive PISTACHIO, BKMS_METABOLIC, PISTACHIO_RINGBREAKER, REAXYS, REAXYS_BIOCATALYSIS database, our tool offers high-accuracy predictions, reflecting the latest in chemical research and data.
Strategy Settings
Precursor scoring | Relevance Heuristic |
---|---|
Min. plausibility | 0.01 |
Model | Template_relevance |
Template Set | Pistachio/Bkms_metabolic/Pistachio_ringbreaker/Reaxys/Reaxys_biocatalysis |
Top-N result to add to graph | 6 |
Feasible Synthetic Routes
体外研究产品的免责声明和信息
请注意,BenchChem 上展示的所有文章和产品信息仅供信息参考。 BenchChem 上可购买的产品专为体外研究设计,这些研究在生物体外进行。体外研究,源自拉丁语 "in glass",涉及在受控实验室环境中使用细胞或组织进行的实验。重要的是要注意,这些产品没有被归类为药物或药品,他们没有得到 FDA 的批准,用于预防、治疗或治愈任何医疗状况、疾病或疾病。我们必须强调,将这些产品以任何形式引入人类或动物的身体都是法律严格禁止的。遵守这些指南对确保研究和实验的法律和道德标准的符合性至关重要。