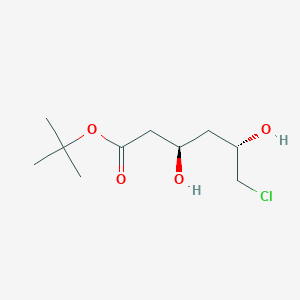
(3R,5S)-叔丁基6-氯-3,5-二羟基己酸酯
描述
Synthesis Analysis
Recent studies have focused on the biosynthesis of "(3R,5S)-tert-Butyl 6-chloro-3,5-dihydroxyhexanoate" using carbonyl reductase from Rhodosporidium toruloides, showing high yields and enantiomeric excess (ee) in mono and biphasic media. The addition of Tween-80 or a water-octanol biphasic system has been shown to enhance the biotransformation process significantly, achieving yields up to 98.9% and ee greater than 99% (Liu et al., 2018). Directed evolution of carbonyl reductase further improved the enzyme's activity, leading to efficient synthesis with high yield and ee (Liu et al., 2017).
科学研究应用
他汀类前体的不对称合成
该化合物用于醛酮还原酶KmAKR不对称合成叔丁基6-氰基-(3R,5R)-二羟基己酸酯,提供了一种绿色方法来生产他汀类前体二手性二醇 (Qiu et al., 2020).
瑞舒伐他汀的关键中间体
它作为瑞舒伐他汀(一种广泛使用的他汀类药物)合成中的关键中间体 (Wang, 2006).
阿托伐他汀钙的构件
该化合物是阿托伐他汀钙(另一种他汀类药物)合成中的重要构件 (Qiu et al., 2019).
使用保加利亚乳杆菌提高产量
使用保加利亚乳杆菌不对称合成该化合物提高了最终产物的浓度和产量 (Amidjojo et al., 2005).
高效的生物合成方法
研究开发了该化合物的有效生物合成方法,包括在单相和双相培养基中使用红酵母属球弗姆的羰基还原酶 (Liu et al., 2018).
化学酶促合成
目前正在研究该化合物所有四个立体异构体的化学酶促合成,突出了其作为1,3-二醇构件的多功能性 (Wolberg et al., 2001).
生物催化工艺开发
各种研究集中于开发合成该化合物的生物催化工艺,包括使用固定化的酿酒酵母和毕赤酵母菌菌株 (Sun et al., 2013) (Yuguo, 2012).
工程酶以提高效率
正在努力提高KmAKR和解淀粉酵母属乳酸醛酮还原酶KlAKR等酶的催化效率,以合成该化合物 (Yu et al., 2019) (Shen et al., 2020).
安全和危害
作用机制
Target of Action
The primary target of (3R,5S)-tert-Butyl 6-chloro-3,5-dihydroxyhexanoate
is the enzyme HMG-CoA reductase . This enzyme plays a crucial role in the mevalonate pathway, which is responsible for the biosynthesis of cholesterol and other isoprenoids.
Mode of Action
The compound (3R,5S)-tert-Butyl 6-chloro-3,5-dihydroxyhexanoate
acts as a competitive inhibitor of HMG-CoA reductase . By binding to the active site of the enzyme, it prevents the conversion of HMG-CoA to mevalonate, a necessary step in cholesterol synthesis.
Biochemical Pathways
The inhibition of HMG-CoA reductase disrupts the mevalonate pathway . This leads to a decrease in the production of cholesterol and other isoprenoids, which are essential components of cell membranes and precursors of various biomolecules.
Pharmacokinetics
The pharmacokinetic properties of (3R,5S)-tert-Butyl 6-chloro-3,5-dihydroxyhexanoate
Its solubility in chloroform and methanol suggests that it may be well-absorbed in the body. Its storage temperature of 2-8°C indicates that it may require specific conditions for optimal stability and bioavailability.
Result of Action
The molecular and cellular effects of (3R,5S)-tert-Butyl 6-chloro-3,5-dihydroxyhexanoate
’s action primarily involve the reduction of cholesterol levels in the body . By inhibiting the synthesis of cholesterol, it can potentially prevent or treat conditions associated with high cholesterol levels, such as atherosclerosis and cardiovascular disease.
Action Environment
Environmental factors can influence the action, efficacy, and stability of (3R,5S)-tert-Butyl 6-chloro-3,5-dihydroxyhexanoate
. For instance, its stability is hygroscopic , meaning it absorbs moisture from the air, which could potentially affect its efficacy and shelf-life. Furthermore, its action may be influenced by factors such as the individual’s metabolic rate, the presence of other drugs, and specific genetic factors.
属性
IUPAC Name |
tert-butyl (3R,5S)-6-chloro-3,5-dihydroxyhexanoate | |
---|---|---|
Source | PubChem | |
URL | https://pubchem.ncbi.nlm.nih.gov | |
Description | Data deposited in or computed by PubChem | |
InChI |
InChI=1S/C10H19ClO4/c1-10(2,3)15-9(14)5-7(12)4-8(13)6-11/h7-8,12-13H,4-6H2,1-3H3/t7-,8+/m1/s1 | |
Source | PubChem | |
URL | https://pubchem.ncbi.nlm.nih.gov | |
Description | Data deposited in or computed by PubChem | |
InChI Key |
FIKPWJZUGTVXCO-SFYZADRCSA-N | |
Source | PubChem | |
URL | https://pubchem.ncbi.nlm.nih.gov | |
Description | Data deposited in or computed by PubChem | |
Canonical SMILES |
CC(C)(C)OC(=O)CC(CC(CCl)O)O | |
Source | PubChem | |
URL | https://pubchem.ncbi.nlm.nih.gov | |
Description | Data deposited in or computed by PubChem | |
Isomeric SMILES |
CC(C)(C)OC(=O)C[C@@H](C[C@@H](CCl)O)O | |
Source | PubChem | |
URL | https://pubchem.ncbi.nlm.nih.gov | |
Description | Data deposited in or computed by PubChem | |
Molecular Formula |
C10H19ClO4 | |
Source | PubChem | |
URL | https://pubchem.ncbi.nlm.nih.gov | |
Description | Data deposited in or computed by PubChem | |
DSSTOX Substance ID |
DTXSID10431205 | |
Record name | tert-Butyl (3R,5S)-6-chloro-3,5-dihydroxyhexanoate | |
Source | EPA DSSTox | |
URL | https://comptox.epa.gov/dashboard/DTXSID10431205 | |
Description | DSSTox provides a high quality public chemistry resource for supporting improved predictive toxicology. | |
Molecular Weight |
238.71 g/mol | |
Source | PubChem | |
URL | https://pubchem.ncbi.nlm.nih.gov | |
Description | Data deposited in or computed by PubChem | |
Product Name |
(3R,5S)-tert-Butyl 6-chloro-3,5-dihydroxyhexanoate | |
CAS RN |
154026-93-4 | |
Record name | tert-Butyl (3R,5S)-6-chloro-3,5-dihydroxyhexanoate | |
Source | EPA DSSTox | |
URL | https://comptox.epa.gov/dashboard/DTXSID10431205 | |
Description | DSSTox provides a high quality public chemistry resource for supporting improved predictive toxicology. | |
Retrosynthesis Analysis
AI-Powered Synthesis Planning: Our tool employs the Template_relevance Pistachio, Template_relevance Bkms_metabolic, Template_relevance Pistachio_ringbreaker, Template_relevance Reaxys, Template_relevance Reaxys_biocatalysis model, leveraging a vast database of chemical reactions to predict feasible synthetic routes.
One-Step Synthesis Focus: Specifically designed for one-step synthesis, it provides concise and direct routes for your target compounds, streamlining the synthesis process.
Accurate Predictions: Utilizing the extensive PISTACHIO, BKMS_METABOLIC, PISTACHIO_RINGBREAKER, REAXYS, REAXYS_BIOCATALYSIS database, our tool offers high-accuracy predictions, reflecting the latest in chemical research and data.
Strategy Settings
Precursor scoring | Relevance Heuristic |
---|---|
Min. plausibility | 0.01 |
Model | Template_relevance |
Template Set | Pistachio/Bkms_metabolic/Pistachio_ringbreaker/Reaxys/Reaxys_biocatalysis |
Top-N result to add to graph | 6 |
Feasible Synthetic Routes
体外研究产品的免责声明和信息
请注意,BenchChem 上展示的所有文章和产品信息仅供信息参考。 BenchChem 上可购买的产品专为体外研究设计,这些研究在生物体外进行。体外研究,源自拉丁语 "in glass",涉及在受控实验室环境中使用细胞或组织进行的实验。重要的是要注意,这些产品没有被归类为药物或药品,他们没有得到 FDA 的批准,用于预防、治疗或治愈任何医疗状况、疾病或疾病。我们必须强调,将这些产品以任何形式引入人类或动物的身体都是法律严格禁止的。遵守这些指南对确保研究和实验的法律和道德标准的符合性至关重要。