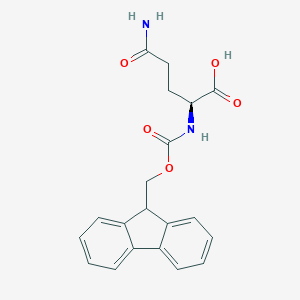
Fmoc-Gln-OH
描述
Fmoc-Gln-OH, also known as 9-fluorenylmethoxycarbonyl-L-glutamine, is a derivative of the amino acid glutamine. It is commonly used in peptide synthesis due to its ability to protect the amino group during the synthesis process. The Fmoc group is a base-labile protecting group, which means it can be removed under basic conditions, making it a popular choice in solid-phase peptide synthesis.
准备方法
Synthetic Routes and Reaction Conditions: The synthesis of Fmoc-Gln-OH typically involves the protection of the amino group of glutamine with the Fmoc group. This can be achieved by reacting glutamine with 9-fluorenylmethoxycarbonyl chloride (Fmoc-Cl) in the presence of a base such as sodium bicarbonate or triethylamine. The reaction is usually carried out in an organic solvent like dimethylformamide (DMF) or dichloromethane (DCM) at room temperature.
Industrial Production Methods: Industrial production of this compound follows similar synthetic routes but on a larger scale. The process involves the use of automated peptide synthesizers that can handle large quantities of reagents and solvents. The reaction conditions are optimized to ensure high yield and purity of the final product. The crude product is then purified using techniques such as high-performance liquid chromatography (HPLC) to remove any impurities.
化学反应分析
Types of Reactions: Fmoc-Gln-OH primarily undergoes deprotection reactions to remove the Fmoc group. This is typically achieved using a base such as piperidine in DMF. The compound can also participate in amide bond formation reactions, which are essential in peptide synthesis.
Common Reagents and Conditions:
Deprotection: Piperidine in DMF is commonly used to remove the Fmoc group.
Amide Bond Formation: Coupling reagents such as N,N’-diisopropylcarbodiimide (DIC) and hydroxybenzotriazole (HOBt) are used to facilitate the formation of amide bonds.
Major Products Formed:
Deprotection: The major product formed is the free amino group of glutamine.
Amide Bond Formation: The major products are peptides with the desired sequence.
科学研究应用
Peptide Synthesis
Fmoc-Gln-OH is primarily utilized in solid-phase peptide synthesis (SPPS) , a widely adopted method for synthesizing peptides. The Fmoc (9-fluorenylmethoxycarbonyl) protecting group allows for mild cleavage conditions, making it preferable over other methods such as Boc (tert-butyloxycarbonyl) chemistry. The advantages of using this compound in SPPS include:
- Milder Reaction Conditions : The cleavage of peptides from the resin can be achieved using trifluoroacetic acid (TFA), which is less hazardous than the liquid hydrogen fluoride used in Boc chemistry. This enhances safety and simplifies the equipment requirements for researchers .
- Compatibility with Modifications : Fmoc chemistry is compatible with the synthesis of peptides that require post-translational modifications, such as phosphorylation and glycosylation, which are often challenging to achieve with Boc methods .
Drug Development
Recent studies have highlighted the role of this compound in drug development, particularly in the synthesis of peptide-based therapeutics. For instance:
- SARS-CoV-2 Protease Substrates : Fmoc-Gln(Trt)-OH has been employed to create peptide substrates for biochemical assays aimed at understanding the substrate specificity of the SARS-CoV-2 main protease (Mpro). This research is crucial for designing inhibitors that could serve as potential treatments for COVID-19 .
- Therapeutic Peptides : The incorporation of this compound into therapeutic peptides has shown promise in enhancing their stability and bioactivity. For example, peptides synthesized using this compound have been evaluated for their efficacy in various biological assays, contributing to the development of novel drugs .
Biochemical Research
In addition to its applications in drug development and peptide synthesis, this compound serves as a valuable tool in biochemical research:
- Substrate Specificity Studies : The compound has been utilized to investigate enzyme-substrate interactions, providing insights into enzyme mechanisms and aiding in the identification of new drug targets .
- Functional Studies : Researchers have used peptides synthesized with this compound to study their biological functions, including their roles in cellular signaling pathways and metabolic processes .
Comparative Properties
The following table summarizes key properties and characteristics of this compound compared to other glutamine derivatives:
Property | This compound | Boc-Gln-OH |
---|---|---|
Cleavage Agent | TFA | HF |
Reaction Conditions | Milder | Harsh |
Compatibility with Modifications | High | Limited |
Epimerization Risk | Lower | Higher |
Case Studies
Several case studies illustrate the practical applications of this compound:
- Study on Peptide Stability : A study demonstrated that peptides synthesized with this compound exhibited higher stability under physiological conditions compared to those synthesized with Boc derivatives. This stability is crucial for therapeutic applications where peptide degradation can lead to loss of efficacy .
- Investigation of Enzyme Activity : Another study utilized this compound to create specific substrates for proteases involved in cancer progression. The findings indicated that these substrates could effectively inhibit protease activity, suggesting potential therapeutic avenues for cancer treatment .
作用机制
The primary mechanism of action of Fmoc-Gln-OH is its role as a protecting group in peptide synthesis. The Fmoc group protects the amino group of glutamine during the synthesis process, preventing unwanted side reactions. The Fmoc group is removed under basic conditions, revealing the free amino group, which can then participate in further reactions to form the desired peptide sequence.
相似化合物的比较
- Fmoc-Ala-OH (9-fluorenylmethoxycarbonyl-L-alanine)
- Fmoc-Glu-OH (9-fluorenylmethoxycarbonyl-L-glutamic acid)
- Fmoc-Lys-OH (9-fluorenylmethoxycarbonyl-L-lysine)
Comparison: Fmoc-Gln-OH is unique in its ability to protect the amino group of glutamine, which is prone to cyclization to form pyroglutamate. This makes it particularly useful in the synthesis of peptides containing glutamine. Other Fmoc-protected amino acids, such as Fmoc-Ala-OH and Fmoc-Glu-OH, serve similar roles in protecting their respective amino groups during peptide synthesis. the choice of Fmoc-protected amino acid depends on the specific requirements of the peptide being synthesized.
生物活性
Fmoc-Gln-OH , or Nα-Fmoc-L-glutamine, is a protected amino acid commonly used in peptide synthesis. Its biological activity is primarily linked to its role as a building block in the formation of peptides and proteins, influencing various biological processes. This article reviews the biological activity of this compound, focusing on its synthesis, applications, and relevant research findings.
Synthesis and Properties
This compound is synthesized through solid-phase peptide synthesis (SPPS), utilizing the Fmoc protection strategy. The Fmoc group facilitates the selective deprotection of the amino acid during peptide assembly. The synthesis involves coupling this compound with other amino acids using coupling reagents such as TBTU (O-(Benzotriazol-1-yl)-N,N,N',N'-tetramethyl uranium tetrafluoroborate) in a controlled environment to ensure high yields and purity .
Biological Applications
- Peptide Synthesis : this compound is extensively used in the synthesis of bioactive peptides. For instance, it has been incorporated into nocistatin-derived peptides, which exhibit significant biological activities, including modulation of neurotransmitter release .
- Enzyme Interaction : Research indicates that peptides containing this compound can interact with various enzymes, influencing their activity. For example, studies have shown that glutamine residues can affect enzyme kinetics and substrate specificity .
- Cell Signaling : Peptides synthesized with this compound have been studied for their roles in cell signaling pathways. They can act as ligands for G protein-coupled receptors (GPCRs), which are crucial for cellular communication and response mechanisms .
Case Study 1: Nocistatin Peptide Synthesis
In a study focused on nocistatin, a hexapeptide containing this compound was synthesized to investigate its effects on neuronal activity. The results demonstrated that nocistatin inhibited synaptic release of neurotransmitters like glycine and GABA, suggesting a potential therapeutic role in neuroprotection .
Case Study 2: Enantioseparation Techniques
A comparative study on enantioseparations of Nα-Fmoc proteinogenic amino acids highlighted the unique retention characteristics of this compound under various chromatographic conditions. The study found that specific mobile phases could enhance the separation efficiency, indicating potential applications in pharmaceutical formulations .
Data Tables
The following table summarizes key properties and applications of this compound based on recent research:
Property | Details |
---|---|
Molecular Formula | C15H18N2O4 |
Molecular Weight | 290.32 g/mol |
Synthesis Method | Solid-phase peptide synthesis (Fmoc strategy) |
Biological Role | Building block in peptide synthesis; influences enzyme activity and signaling |
Applications | Neuroactive peptides, enzyme modulators, pharmaceutical development |
属性
IUPAC Name |
(2S)-5-amino-2-(9H-fluoren-9-ylmethoxycarbonylamino)-5-oxopentanoic acid | |
---|---|---|
Source | PubChem | |
URL | https://pubchem.ncbi.nlm.nih.gov | |
Description | Data deposited in or computed by PubChem | |
InChI |
InChI=1S/C20H20N2O5/c21-18(23)10-9-17(19(24)25)22-20(26)27-11-16-14-7-3-1-5-12(14)13-6-2-4-8-15(13)16/h1-8,16-17H,9-11H2,(H2,21,23)(H,22,26)(H,24,25)/t17-/m0/s1 | |
Source | PubChem | |
URL | https://pubchem.ncbi.nlm.nih.gov | |
Description | Data deposited in or computed by PubChem | |
InChI Key |
IZKGGDFLLNVXNZ-KRWDZBQOSA-N | |
Source | PubChem | |
URL | https://pubchem.ncbi.nlm.nih.gov | |
Description | Data deposited in or computed by PubChem | |
Canonical SMILES |
C1=CC=C2C(=C1)C(C3=CC=CC=C32)COC(=O)NC(CCC(=O)N)C(=O)O | |
Source | PubChem | |
URL | https://pubchem.ncbi.nlm.nih.gov | |
Description | Data deposited in or computed by PubChem | |
Isomeric SMILES |
C1=CC=C2C(=C1)C(C3=CC=CC=C32)COC(=O)N[C@@H](CCC(=O)N)C(=O)O | |
Source | PubChem | |
URL | https://pubchem.ncbi.nlm.nih.gov | |
Description | Data deposited in or computed by PubChem | |
Molecular Formula |
C20H20N2O5 | |
Source | PubChem | |
URL | https://pubchem.ncbi.nlm.nih.gov | |
Description | Data deposited in or computed by PubChem | |
DSSTOX Substance ID |
DTXSID40922737 | |
Record name | N-{[(9H-Fluoren-9-yl)methoxy](hydroxy)methylidene}-5-hydroxy-5-iminonorvaline | |
Source | EPA DSSTox | |
URL | https://comptox.epa.gov/dashboard/DTXSID40922737 | |
Description | DSSTox provides a high quality public chemistry resource for supporting improved predictive toxicology. | |
Molecular Weight |
368.4 g/mol | |
Source | PubChem | |
URL | https://pubchem.ncbi.nlm.nih.gov | |
Description | Data deposited in or computed by PubChem | |
CAS No. |
71989-20-3, 118609-68-0 | |
Record name | N2-(9-Fluorenylmethoxycarbonyl)-L-glutamine | |
Source | CAS Common Chemistry | |
URL | https://commonchemistry.cas.org/detail?cas_rn=71989-20-3 | |
Description | CAS Common Chemistry is an open community resource for accessing chemical information. Nearly 500,000 chemical substances from CAS REGISTRY cover areas of community interest, including common and frequently regulated chemicals, and those relevant to high school and undergraduate chemistry classes. This chemical information, curated by our expert scientists, is provided in alignment with our mission as a division of the American Chemical Society. | |
Explanation | The data from CAS Common Chemistry is provided under a CC-BY-NC 4.0 license, unless otherwise stated. | |
Record name | N-{[(9H-Fluoren-9-yl)methoxy](hydroxy)methylidene}-5-hydroxy-5-iminonorvaline | |
Source | EPA DSSTox | |
URL | https://comptox.epa.gov/dashboard/DTXSID40922737 | |
Description | DSSTox provides a high quality public chemistry resource for supporting improved predictive toxicology. | |
Record name | N2-[(9H-fluoren-9-ylmethoxy)carbonyl]-L-glutamine | |
Source | European Chemicals Agency (ECHA) | |
URL | https://echa.europa.eu/substance-information/-/substanceinfo/100.069.301 | |
Description | The European Chemicals Agency (ECHA) is an agency of the European Union which is the driving force among regulatory authorities in implementing the EU's groundbreaking chemicals legislation for the benefit of human health and the environment as well as for innovation and competitiveness. | |
Explanation | Use of the information, documents and data from the ECHA website is subject to the terms and conditions of this Legal Notice, and subject to other binding limitations provided for under applicable law, the information, documents and data made available on the ECHA website may be reproduced, distributed and/or used, totally or in part, for non-commercial purposes provided that ECHA is acknowledged as the source: "Source: European Chemicals Agency, http://echa.europa.eu/". Such acknowledgement must be included in each copy of the material. ECHA permits and encourages organisations and individuals to create links to the ECHA website under the following cumulative conditions: Links can only be made to webpages that provide a link to the Legal Notice page. | |
Retrosynthesis Analysis
AI-Powered Synthesis Planning: Our tool employs the Template_relevance Pistachio, Template_relevance Bkms_metabolic, Template_relevance Pistachio_ringbreaker, Template_relevance Reaxys, Template_relevance Reaxys_biocatalysis model, leveraging a vast database of chemical reactions to predict feasible synthetic routes.
One-Step Synthesis Focus: Specifically designed for one-step synthesis, it provides concise and direct routes for your target compounds, streamlining the synthesis process.
Accurate Predictions: Utilizing the extensive PISTACHIO, BKMS_METABOLIC, PISTACHIO_RINGBREAKER, REAXYS, REAXYS_BIOCATALYSIS database, our tool offers high-accuracy predictions, reflecting the latest in chemical research and data.
Strategy Settings
Precursor scoring | Relevance Heuristic |
---|---|
Min. plausibility | 0.01 |
Model | Template_relevance |
Template Set | Pistachio/Bkms_metabolic/Pistachio_ringbreaker/Reaxys/Reaxys_biocatalysis |
Top-N result to add to graph | 6 |
Feasible Synthetic Routes
体外研究产品的免责声明和信息
请注意,BenchChem 上展示的所有文章和产品信息仅供信息参考。 BenchChem 上可购买的产品专为体外研究设计,这些研究在生物体外进行。体外研究,源自拉丁语 "in glass",涉及在受控实验室环境中使用细胞或组织进行的实验。重要的是要注意,这些产品没有被归类为药物或药品,他们没有得到 FDA 的批准,用于预防、治疗或治愈任何医疗状况、疾病或疾病。我们必须强调,将这些产品以任何形式引入人类或动物的身体都是法律严格禁止的。遵守这些指南对确保研究和实验的法律和道德标准的符合性至关重要。