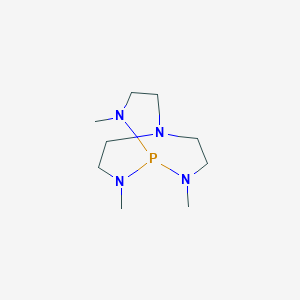
丙氮磷杂环烷
描述
2,8,9-Trimethyl-2,5,8,9-tetraaza-1-phosphabicyclo[3.3.3]undecane is a highly versatile compound known for its strong basic properties. It is often referred to as a superbase due to its exceptional ability to deprotonate weak acids. This compound is widely used in various chemical reactions and industrial applications due to its unique structure and reactivity .
科学研究应用
2,8,9-Trimethyl-2,5,8,9-tetraaza-1-phosphabicyclo[3.3.3]undecane has a wide range of scientific research applications, including:
作用机制
Target of Action
The primary targets of Proazaphosphatrane are organic isocyanates . It is known to efficiently catalyse the cyclo-trimerisation of these organic isocyanates . Additionally, it has been reported that Proazaphosphatrane can interact with metal centers, such as rhodium .
Mode of Action
Proazaphosphatrane interacts with its targets through a process known as transannulation . This process involves an intramolecular interaction between the axial nitrogen and phosphorus upon binding to an electrophile . In the case of organic isocyanates, Proazaphosphatrane catalyses their cyclo-trimerisation, leading to the formation of high-purity isocyanurate with little uretdione by-product .
Biochemical Pathways
The primary biochemical pathway affected by Proazaphosphatrane is the cyclo-trimerisation of organic isocyanates . This process is vital in the polymerisation process when tailoring the mechanical properties of materials like polyurethane foams . The cyclo-trimerisation leads to the formation of high-purity isocyanurate .
Result of Action
The result of Proazaphosphatrane’s action is the formation of high-purity isocyanurate from the cyclo-trimerisation of organic isocyanates . This process is highly efficient, producing little uretdione by-product . This makes Proazaphosphatrane an effective catalyst for this reaction, which is crucial in the production of materials like polyurethane foams .
Action Environment
The action of Proazaphosphatrane can be influenced by environmental factors. For instance, the presence of a Lewis acid, such as lithium chloride, can promote the dynamic behavior of Proazaphosphatrane in solution . Moreover, Proazaphosphatrane is tolerant of water within the reaction medium, with a beneficial and cooperative effect being observed .
生化分析
Biochemical Properties
Proazaphosphatrane is known to efficiently catalyse the cyclo-trimerisation of organic isocyanates, giving high purity isocyanurate with little uretdione by-product . This biochemical reaction is crucial in the formation of polyurethane foams, which are widely used materials .
Cellular Effects
Given its role in the synthesis of polyurethane foams, it can be inferred that it may have significant effects on cellular processes involving organic isocyanates .
Molecular Mechanism
The molecular mechanism of Proazaphosphatrane involves a nucleophilic-catalysis reaction pathway involving sequential addition of methyl isocyanate to activated zwitterionic intermediates . Evidence for significant transannulation by the Proazaphosphatrane nitrogen was found for all intermediates, offering stabilisation of the phosphonium cation . Steric crowding at the Proazaphosphatrane nucleophilic phosphorus gives rise to a preference for direct isocyanurate formation rather than via the uretdione .
Temporal Effects in Laboratory Settings
It is known that Proazaphosphatrane can undergo structural deformations due to transannulation .
Metabolic Pathways
It is known that Proazaphosphatrane can efficiently catalyse the cyclo-trimerisation of organic isocyanates .
准备方法
Synthetic Routes and Reaction Conditions
The synthesis of 2,8,9-Trimethyl-2,5,8,9-tetraaza-1-phosphabicyclo[3.3.3]undecane typically involves the reaction of tris(2-aminoethyl)amine with phosphorus trichloride, followed by methylation. The reaction conditions often require an inert atmosphere and controlled temperatures to ensure the desired product is obtained .
Industrial Production Methods
In industrial settings, the production of this compound may involve large-scale reactors and continuous flow processes to optimize yield and purity. The use of advanced purification techniques, such as distillation and crystallization, is common to achieve high-quality products .
化学反应分析
Types of Reactions
2,8,9-Trimethyl-2,5,8,9-tetraaza-1-phosphabicyclo[3.3.3]undecane undergoes various types of chemical reactions, including:
Oxidation: It can be oxidized to form phosphine oxides.
Reduction: It can be reduced to form phosphines.
Substitution: It participates in nucleophilic substitution reactions, particularly with halides.
Common Reagents and Conditions
Common reagents used in reactions with this compound include halides, oxidizing agents, and reducing agents. Typical reaction conditions involve the use of solvents such as dichloromethane or toluene, and reactions are often carried out under an inert atmosphere to prevent unwanted side reactions .
Major Products Formed
The major products formed from reactions involving 2,8,9-Trimethyl-2,5,8,9-tetraaza-1-phosphabicyclo[3.3.3]undecane include phosphine oxides, phosphines, and various substituted derivatives depending on the specific reagents and conditions used .
相似化合物的比较
Similar Compounds
2,8,9-Triisobutyl-2,5,8,9-tetraaza-1-phosphabicyclo[3.3.3]undecane: Similar in structure but with isobutyl groups instead of methyl groups.
2,8,9-Triisopropyl-2,5,8,9-tetraaza-1-phosphabicyclo[3.3.3]undecane: Similar in structure but with isopropyl groups instead of methyl groups.
Phosphazene base P1-t-Bu: Another strong base with a different structural framework.
Uniqueness
The uniqueness of 2,8,9-Trimethyl-2,5,8,9-tetraaza-1-phosphabicyclo[3.3.3]undecane lies in its exceptional basicity and stability, making it a valuable reagent in both laboratory and industrial settings. Its ability to participate in a wide range of chemical reactions and its effectiveness as a catalyst set it apart from other similar compounds .
属性
IUPAC Name |
2,8,9-trimethyl-2,5,8,9-tetraza-1-phosphabicyclo[3.3.3]undecane | |
---|---|---|
Source | PubChem | |
URL | https://pubchem.ncbi.nlm.nih.gov | |
Description | Data deposited in or computed by PubChem | |
InChI |
InChI=1S/C9H21N4P/c1-10-4-7-13-8-5-11(2)14(10)12(3)6-9-13/h4-9H2,1-3H3 | |
Source | PubChem | |
URL | https://pubchem.ncbi.nlm.nih.gov | |
Description | Data deposited in or computed by PubChem | |
InChI Key |
PCYSWBQHCWWSFW-UHFFFAOYSA-N | |
Source | PubChem | |
URL | https://pubchem.ncbi.nlm.nih.gov | |
Description | Data deposited in or computed by PubChem | |
Canonical SMILES |
CN1CCN2CCN(P1N(CC2)C)C | |
Source | PubChem | |
URL | https://pubchem.ncbi.nlm.nih.gov | |
Description | Data deposited in or computed by PubChem | |
Molecular Formula |
C9H21N4P | |
Source | PubChem | |
URL | https://pubchem.ncbi.nlm.nih.gov | |
Description | Data deposited in or computed by PubChem | |
DSSTOX Substance ID |
DTXSID90400494 | |
Record name | Verkade base | |
Source | EPA DSSTox | |
URL | https://comptox.epa.gov/dashboard/DTXSID90400494 | |
Description | DSSTox provides a high quality public chemistry resource for supporting improved predictive toxicology. | |
Molecular Weight |
216.26 g/mol | |
Source | PubChem | |
URL | https://pubchem.ncbi.nlm.nih.gov | |
Description | Data deposited in or computed by PubChem | |
CAS No. |
120666-13-9 | |
Record name | 2,8,9-Trimethyl-2,5,8,9-tetraaza-1-phosphabicyclo[3.3.3]undecane | |
Source | CAS Common Chemistry | |
URL | https://commonchemistry.cas.org/detail?cas_rn=120666-13-9 | |
Description | CAS Common Chemistry is an open community resource for accessing chemical information. Nearly 500,000 chemical substances from CAS REGISTRY cover areas of community interest, including common and frequently regulated chemicals, and those relevant to high school and undergraduate chemistry classes. This chemical information, curated by our expert scientists, is provided in alignment with our mission as a division of the American Chemical Society. | |
Explanation | The data from CAS Common Chemistry is provided under a CC-BY-NC 4.0 license, unless otherwise stated. | |
Record name | Proazaphosphatrane | |
Source | ChemIDplus | |
URL | https://pubchem.ncbi.nlm.nih.gov/substance/?source=chemidplus&sourceid=0120666139 | |
Description | ChemIDplus is a free, web search system that provides access to the structure and nomenclature authority files used for the identification of chemical substances cited in National Library of Medicine (NLM) databases, including the TOXNET system. | |
Record name | Verkade base | |
Source | EPA DSSTox | |
URL | https://comptox.epa.gov/dashboard/DTXSID90400494 | |
Description | DSSTox provides a high quality public chemistry resource for supporting improved predictive toxicology. | |
Record name | PROAZAPHOSPHATRANE | |
Source | FDA Global Substance Registration System (GSRS) | |
URL | https://gsrs.ncats.nih.gov/ginas/app/beta/substances/LK9MJR52XE | |
Description | The FDA Global Substance Registration System (GSRS) enables the efficient and accurate exchange of information on what substances are in regulated products. Instead of relying on names, which vary across regulatory domains, countries, and regions, the GSRS knowledge base makes it possible for substances to be defined by standardized, scientific descriptions. | |
Explanation | Unless otherwise noted, the contents of the FDA website (www.fda.gov), both text and graphics, are not copyrighted. They are in the public domain and may be republished, reprinted and otherwise used freely by anyone without the need to obtain permission from FDA. Credit to the U.S. Food and Drug Administration as the source is appreciated but not required. | |
Q1: What are proazaphosphatranes?
A1: Proazaphosphatranes, also known as Verkade's superbases, are a class of bicyclic phosphorus compounds known for their exceptional basicity. They are characterized by a transannular P-N interaction, which enhances their electron-donating ability. [, , , ]
Q2: What is the structural formula and molecular weight of a common proazaphosphatrane?
A2: A widely studied example is 2,8,9-tri-i-butyl-2,5,8,9-tetraaza-1-phosphabicyclo[3.3.3]undecane, also known as P(i-BuNCH2CH2)3N. It has a molecular weight of 342.50 g/mol. []
Q3: How do proazaphosphatranes achieve their superbasicity?
A3: Their exceptional basicity stems from the combination of a pyramidal phosphorus atom with three electron-donating nitrogen atoms and the unique transannular P-N interaction. This interaction allows for increased electron density on the phosphorus atom, making it highly nucleophilic and capable of abstracting protons even from weakly acidic compounds. [, , ]
Q4: What is transannulation, and how does it influence proazaphosphatrane properties?
A4: Transannulation refers to the interaction between the phosphorus atom and the nitrogen atom positioned across the bicyclic ring system in proazaphosphatranes. This interaction, influenced by the substituents on the phosphorus and nitrogen atoms, modulates the P-N bond distance and electron density at the phosphorus center. This directly affects the proazaphosphatrane's basicity, donor strength, and reactivity. [, , ]
Q5: How does the structure of proazaphosphatranes relate to their catalytic activity?
A5: The transannular P-N bond plays a crucial role in their catalytic activity. Upon interaction with an electrophile, this bond can elongate, allowing the phosphorus atom to form a strong bond with the electrophilic center of the substrate, thus activating it for further reactions. [, , ]
Q6: What types of reactions can proazaphosphatranes catalyze?
A6: Proazaphosphatranes are versatile catalysts, effectively facilitating a wide array of reactions including:
- C-N bond formations: Notably, they serve as ligands in palladium-catalyzed Buchwald-Hartwig amination reactions, allowing the coupling of aryl chlorides with various amines. [, , , ]
- Stille cross-coupling: They effectively catalyze the coupling of aryl and vinyl chlorides with organotin reagents, enabling the synthesis of various organic compounds. []
- Isomerization reactions: Proazaphosphatranes are capable of catalyzing the isomerization of allylic systems and methylene-interrupted dienes, providing valuable tools for alkene synthesis. [, ]
- Cyclotrimerization of isocyanates: They act as efficient catalysts for the cyclotrimerization of isocyanates, yielding isocyanurates with high purity, a crucial process in polyurethane synthesis. [, ]
- Group transfer polymerization: They can catalyze the controlled polymerization of methyl methacrylate, leading to the formation of poly(methyl methacrylate) with specific molecular weights and narrow polydispersities. []
Q7: Are there any limitations to using proazaphosphatranes as catalysts?
A7: While highly effective catalysts, proazaphosphatranes exhibit sensitivity to moisture and air. Proper handling and storage under inert conditions are crucial for maintaining their reactivity. []
Q8: Can proazaphosphatranes be used as ligands in transition metal complexes?
A9: Yes, proazaphosphatranes can act as ligands for transition metals. For instance, they form complexes with palladium, which are highly active in cross-coupling reactions like the Stille and Buchwald-Hartwig aminations. The strong sigma-donating ability of proazaphosphatranes enhances the reactivity of the metal center, enabling challenging bond formations. [, , , , ]
Q9: What factors influence the donor strength of proazaphosphatranes when complexed with transition metals?
A10: The electron-donating ability of proazaphosphatranes can be modulated by the nature of the transition metal center and its oxidation state. More electron-deficient metal ions, or those in higher oxidation states, induce a greater degree of transannular interaction in the proazaphosphatrane ligand. This results in a stronger P-M bond and a more electron-rich metal center. []
Q10: Can proazaphosphatranes be modified to tune their properties?
A11: Yes, the properties of proazaphosphatranes can be fine-tuned through structural modifications. Changing the substituents on the nitrogen atoms of the proazaphosphatrane framework significantly influences its basicity, steric hindrance, and consequently, its reactivity and catalytic activity. [, , , ]
Q11: What are some examples of modified proazaphosphatranes, and how do their properties differ?
A12: Researchers have synthesized various proazaphosphatrane derivatives with varying steric and electronic properties. For example, proazaphosphatranes with bulky substituents like i-Bu groups show enhanced catalytic activity in some reactions due to increased steric hindrance. Conversely, electron-donating substituents on the nitrogen atoms can further enhance the basicity of the proazaphosphatrane. [, , , , , ]
Q12: How does computational chemistry contribute to understanding proazaphosphatrane chemistry?
A12: Computational methods like Density Functional Theory (DFT) have been instrumental in elucidating the unique properties of proazaphosphatranes. These calculations provide valuable insights into:
- Design of new catalysts: By simulating the properties of various proazaphosphatrane derivatives, computational chemistry aids in the rational design of new catalysts with improved activity, selectivity, and stability. [, , , ]
Q13: Have proazaphosphatranes been investigated for their potential in enantioselective catalysis?
A14: Yes, the development of chiral proazaphosphatranes for asymmetric synthesis is an active area of research. Researchers have synthesized proazaphosphatranes with chiral substituents on the nitrogen atoms, aiming to induce enantioselectivity in various reactions. These chiral proazaphosphatranes hold promise for the synthesis of enantiomerically enriched compounds, which are highly valuable in pharmaceutical and agrochemical industries. []
Q14: What are azaphosphatranes, and how are they related to proazaphosphatranes?
A15: Azaphosphatranes are the conjugate acids of proazaphosphatranes. They are formed upon protonation of the proazaphosphatrane, resulting in a formal positive charge on the phosphorus atom. Although less explored than their proazaphosphatrane counterparts, azaphosphatranes have also shown potential as catalysts in specific reactions. [, , ]
Q15: Can azaphosphatranes be used as catalysts? What are their advantages?
A16: While less basic than proazaphosphatranes, azaphosphatranes demonstrate catalytic activity in various reactions, including the synthesis of cyclic carbonates from epoxides and carbon dioxide. Their robustness and hydrophobicity make them attractive alternatives to proazaphosphatranes in reactions sensitive to strong bases. [, ]
Q16: What is the significance of studying proazaphosphatranes encapsulated in confined spaces?
A16: Encapsulating proazaphosphatranes within molecular cages or nanopores offers intriguing opportunities to control their reactivity and selectivity. The confined environment can:
- Enhance selectivity: The confined space can impose steric constraints on the reaction, leading to improved diastereoselectivity or enantioselectivity in the product formation. [, , ]
Q17: What are some examples of confined proazaphosphatrane systems, and what advantages do they offer?
A18: Hemicryptophanes, a type of molecular cage, have been used to encapsulate proazaphosphatranes. These systems have demonstrated enhanced diastereoselectivity in Diels-Alder reactions compared to their non-encapsulated counterparts. Additionally, immobilizing azaphosphatranes within the nanopores of mesoporous silica materials provides recyclable heterogeneous catalysts for various organic transformations. [, , ]
体外研究产品的免责声明和信息
请注意,BenchChem 上展示的所有文章和产品信息仅供信息参考。 BenchChem 上可购买的产品专为体外研究设计,这些研究在生物体外进行。体外研究,源自拉丁语 "in glass",涉及在受控实验室环境中使用细胞或组织进行的实验。重要的是要注意,这些产品没有被归类为药物或药品,他们没有得到 FDA 的批准,用于预防、治疗或治愈任何医疗状况、疾病或疾病。我们必须强调,将这些产品以任何形式引入人类或动物的身体都是法律严格禁止的。遵守这些指南对确保研究和实验的法律和道德标准的符合性至关重要。