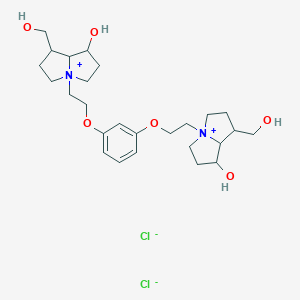
地柏素
描述
Diplacin is a complex compound with specific synthesis methods and unique molecular characteristics. It has distinct chemical and physical properties, making it a subject of interest in organic chemistry.
Synthesis Analysis
- Synthesis of Diplacin: The synthesis process for compounds similar to Diplacin often involves complex chemical reactions. For example, the synthesis of cyclic tripeptides uses intramolecular Sonogashira coupling or macrolactamization, yielding rigidified peptidic macrocycles (Brink, Rijkers, & Liskamp, 2006).
Molecular Structure Analysis
- Characterization of Molecular Structure: Detailed molecular structure analysis is crucial for understanding the properties of Diplacin. Studies on similar compounds have used NMR spectroscopy for conformational analysis, as in the case of microcin SF608 (Valls, Vallribera, López-Canet, & Bonjoch, 2002).
Chemical Reactions and Properties
- Chemical Reactions: The reactivity of Diplacin can be inferred from studies on similar compounds. For instance, the synthesis of microcin SF608 involved coupling reactions and deprotection steps (Valls et al., 2002).
- Chemical Properties: Chemical properties are often determined by the molecular structure and the types of chemical bonds in the compound. Research on similar compounds provides insights into how molecular structure influences chemical behavior (Nagao, 2002).
Physical Properties Analysis
- Physical Properties: The physical properties of Diplacin, like solubility, melting point, and stability, can be extrapolated from related compounds. For instance, the study on microcapsulated capsaicin using diphase emulsion method revealed improved melting point and thermal stability, showcasing how encapsulation can modify physical properties (Wang, Chen, Xu, Xue, & Lou, 2011).
Chemical Properties Analysis
- Chemical Stability and Reactivity: Understanding the chemical stability and reactivity of Diplacin requires a comprehensive analysis of its molecular structure and the nature of its chemical bonds, as demonstrated in similar compounds (Carey, Laffan, Thomson, & Williams, 2006).
科学研究应用
抗炎特性:地柏素的成分地柏酮已显示出调节炎症反应的能力。它下调促炎因子,如肿瘤坏死因子α和单核细胞趋化蛋白1,同时上调抗炎蛋白,如锌指蛋白36。这表明其在治疗炎症性疾病中的潜力(Hošek, Závalová, Šmejkal, & Bartoš,2010)。
与氢化可的松的相互作用:在一项对幼鼠的研究中,发现地柏素可以减轻氢化可的松对身体发育的影响,表明其对糖皮质激素作用具有调节作用(Nezhentsev,1980)。
抗菌活性:与地柏素相关的植物物种地柏的提取物对多种人畜致病菌如大肠杆菌、沙门氏菌属和金黄色葡萄球菌表现出有效性。这表明其在抗菌应用方面的潜力(Semwal, Sunil, Bhattacharya, & Arvind,2011)。
神经影响:在地柏素致敏的狗中观察到地柏素对肌肉收缩和骨骼肌中的过敏反应具有箭毒样作用,这表明其在调节肌肉和过敏反应中发挥作用(Rakhmatullin,2004)。
对血化学的影响:发现用地柏素和其他糖皮质激素治疗14天会增加血液中高铁血红蛋白的含量,表明其对血化学的潜在影响(Livshin, Averburg, & Nezhentsev,1983)。
癌症治疗潜力:发现地柏素(以双嘧达莫的形式)可以增强阿霉素对癌细胞的细胞毒性作用,表明其在增强化疗疗效中发挥作用(Kusumoto, Maehara, Anai, Kusumoto, & Sugimachi,1988)。
视觉皮层活动:研究表明,地柏素会影响猫视觉皮层神经元的活动,特别是对血清素和乙酰胆碱的反应。这表明其对感觉处理的潜在影响(Perkhurova,2005); (Perkhurova & Zarkeshev,1977)。
药物性肺炎管理:研究强调了雷帕霉素(mTOR)抑制剂在管理癌症患者药物性肺炎中的作用,其中包括地柏素等化合物,强调了早期识别和干预的重要性(Durán等人,2014)。
属性
IUPAC Name |
4-[2-[3-[2-[1-hydroxy-7-(hydroxymethyl)-2,3,5,6,7,8-hexahydro-1H-pyrrolizin-4-ium-4-yl]ethoxy]phenoxy]ethyl]-7-(hydroxymethyl)-2,3,5,6,7,8-hexahydro-1H-pyrrolizin-4-ium-1-ol;dichloride | |
---|---|---|
Source | PubChem | |
URL | https://pubchem.ncbi.nlm.nih.gov | |
Description | Data deposited in or computed by PubChem | |
InChI |
InChI=1S/C26H42N2O6.2ClH/c29-17-19-4-8-27(10-6-23(31)25(19)27)12-14-33-21-2-1-3-22(16-21)34-15-13-28-9-5-20(18-30)26(28)24(32)7-11-28;;/h1-3,16,19-20,23-26,29-32H,4-15,17-18H2;2*1H/q+2;;/p-2 | |
Source | PubChem | |
URL | https://pubchem.ncbi.nlm.nih.gov | |
Description | Data deposited in or computed by PubChem | |
InChI Key |
RUNACLFUDAJMTR-UHFFFAOYSA-L | |
Source | PubChem | |
URL | https://pubchem.ncbi.nlm.nih.gov | |
Description | Data deposited in or computed by PubChem | |
Canonical SMILES |
C1C[N+]2(CCC(C2C1CO)O)CCOC3=CC(=CC=C3)OCC[N+]45CCC(C4C(CC5)O)CO.[Cl-].[Cl-] | |
Source | PubChem | |
URL | https://pubchem.ncbi.nlm.nih.gov | |
Description | Data deposited in or computed by PubChem | |
Molecular Formula |
C26H42Cl2N2O6 | |
Source | PubChem | |
URL | https://pubchem.ncbi.nlm.nih.gov | |
Description | Data deposited in or computed by PubChem | |
DSSTOX Substance ID |
DTXSID90941832 | |
Record name | 4,4'-[1,3-Phenylenebis(oxyethane-2,1-diyl)]bis[1-hydroxy-7-(hydroxymethyl)hexahydro-1H-pyrrolizin-4-ium] dichloride | |
Source | EPA DSSTox | |
URL | https://comptox.epa.gov/dashboard/DTXSID90941832 | |
Description | DSSTox provides a high quality public chemistry resource for supporting improved predictive toxicology. | |
Molecular Weight |
549.5 g/mol | |
Source | PubChem | |
URL | https://pubchem.ncbi.nlm.nih.gov | |
Description | Data deposited in or computed by PubChem | |
Product Name |
Diplacin | |
CAS RN |
19918-85-5 | |
Record name | Diplacin | |
Source | ChemIDplus | |
URL | https://pubchem.ncbi.nlm.nih.gov/substance/?source=chemidplus&sourceid=0019918855 | |
Description | ChemIDplus is a free, web search system that provides access to the structure and nomenclature authority files used for the identification of chemical substances cited in National Library of Medicine (NLM) databases, including the TOXNET system. | |
Record name | 4,4'-[1,3-Phenylenebis(oxyethane-2,1-diyl)]bis[1-hydroxy-7-(hydroxymethyl)hexahydro-1H-pyrrolizin-4-ium] dichloride | |
Source | EPA DSSTox | |
URL | https://comptox.epa.gov/dashboard/DTXSID90941832 | |
Description | DSSTox provides a high quality public chemistry resource for supporting improved predictive toxicology. | |
Retrosynthesis Analysis
AI-Powered Synthesis Planning: Our tool employs the Template_relevance Pistachio, Template_relevance Bkms_metabolic, Template_relevance Pistachio_ringbreaker, Template_relevance Reaxys, Template_relevance Reaxys_biocatalysis model, leveraging a vast database of chemical reactions to predict feasible synthetic routes.
One-Step Synthesis Focus: Specifically designed for one-step synthesis, it provides concise and direct routes for your target compounds, streamlining the synthesis process.
Accurate Predictions: Utilizing the extensive PISTACHIO, BKMS_METABOLIC, PISTACHIO_RINGBREAKER, REAXYS, REAXYS_BIOCATALYSIS database, our tool offers high-accuracy predictions, reflecting the latest in chemical research and data.
Strategy Settings
Precursor scoring | Relevance Heuristic |
---|---|
Min. plausibility | 0.01 |
Model | Template_relevance |
Template Set | Pistachio/Bkms_metabolic/Pistachio_ringbreaker/Reaxys/Reaxys_biocatalysis |
Top-N result to add to graph | 6 |
Feasible Synthetic Routes
Q & A
Q1: What is Diplacin and what is its primary mechanism of action?
A1: Diplacin is a non-depolarizing neuromuscular blocking agent. It acts as a competitive antagonist at nicotinic acetylcholine receptors at the neuromuscular junction. [, , , , ] This prevents acetylcholine from binding to its receptors, inhibiting muscle contraction and leading to muscle relaxation or paralysis.
Q2: How does Diplacin's effect differ from depolarizing muscle relaxants?
A2: Unlike depolarizing muscle relaxants like succinylcholine, which initially activate the acetylcholine receptor before blocking it, Diplacin does not cause depolarization. It directly blocks the receptor, resulting in a more predictable and easily reversible paralysis. [, ]
Q3: What are the downstream effects of Diplacin administration?
A3: Diplacin administration leads to skeletal muscle relaxation. This effect is utilized clinically for surgical procedures, particularly to facilitate endotracheal intubation and mechanical ventilation. [, , ]
Q4: Can Diplacin cross the blood-brain barrier?
A4: Diplacin does not readily cross the blood-brain barrier. [, , ] Consequently, it primarily exerts its effects peripherally and does not significantly impact the central nervous system.
Q5: What is the molecular formula and weight of Diplacin?
A5: Unfortunately, the provided research abstracts do not specify the molecular formula and weight of Diplacin. Further investigation into its chemical structure is needed.
Q6: What is known about the stability of Diplacin in different formulations?
A6: The research abstracts primarily focus on Diplacin's pharmacological effects and do not provide information about its stability or formulation strategies.
Q7: What are the pharmacokinetic properties of Diplacin?
A8: While the abstracts do not offer a detailed pharmacokinetic profile, they indicate that Diplacin is administered intravenously [, ] and its effects are relatively short-lived. [] Further studies are needed to elucidate its absorption, distribution, metabolism, and excretion characteristics.
Q8: What factors can influence the pharmacodynamics of Diplacin?
A9: The research suggests that the hypothermia stage can influence the action of Diplacin on the hypothermia development ratio (HDR) in rats. [] Further research is necessary to fully understand the factors modulating its pharmacodynamic profile.
Q9: What experimental models have been used to study Diplacin's effects?
A10: The research highlights the use of in vitro and in vivo models to investigate Diplacin's actions. Studies employed rat models to assess its impact on erythrocyte resistance to hypoosmotic hemolysis [, ], growth and development in rat pups [, ], thermoregulation [, ], methemoglobin formation [], and its interaction with narcotics. [, ] Additionally, cat models were used to examine its effects on transcallosal potentials in the sensomotor cortex [, ] and the activities of the cortical portion of the visual analyzer. [, ]
Q10: Has Diplacin been studied in clinical trials?
A11: While the provided research doesn't cite specific clinical trials, some abstracts mention the clinical application of Diplacin in surgery, particularly its use in anesthesia for endotracheal intubation. [, , ]
Q11: Has Diplacin been investigated for its potential in managing spasticity?
A13: Yes, research has explored the use of Diplacin for treating spasticity caused by spinal trauma. [, ] This area requires further exploration to determine its efficacy and safety in managing spasticity.
体外研究产品的免责声明和信息
请注意,BenchChem 上展示的所有文章和产品信息仅供信息参考。 BenchChem 上可购买的产品专为体外研究设计,这些研究在生物体外进行。体外研究,源自拉丁语 "in glass",涉及在受控实验室环境中使用细胞或组织进行的实验。重要的是要注意,这些产品没有被归类为药物或药品,他们没有得到 FDA 的批准,用于预防、治疗或治愈任何医疗状况、疾病或疾病。我们必须强调,将这些产品以任何形式引入人类或动物的身体都是法律严格禁止的。遵守这些指南对确保研究和实验的法律和道德标准的符合性至关重要。