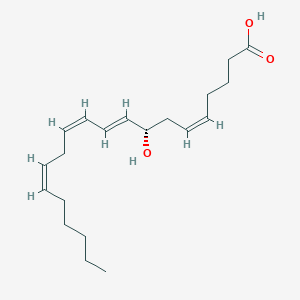
8S-HETE
描述
8(S)-HETE is a HETE having an (8S)-hydroxy group and (5Z)-, (9E)-, (11Z)- and (14Z)-double bonds. It is functionally related to an icosa-5,9,11,14-tetraenoic acid. It is a conjugate acid of an 8(S)-HETE(1-). It is an enantiomer of an 8(R)-HETE.
科学研究应用
在表皮肿瘤发展中的作用
8S-HETE是8S脂氧合酶(8SLOX)的产物,在乳头状瘤的表皮肿瘤发展中起着重要作用,并诱导基底角质形成细胞的染色体改变 . 这表明this compound可能是皮肤癌治疗干预的潜在靶点。
参与炎症性皮肤病
观察到this compound在炎症性皮肤病中上调 . 这表明它可能参与了这些疾病的发病机制,为治疗靶点提供了另一个途径。
8S脂氧合酶的潜在抑制剂
发现某些来自药用植物的生物活性化合物可以抑制产生this compound的8S LOX蛋白 . 这些化合物可能被开发成用于治疗牛皮癣等炎症性皮肤病的药物 .
生物活性代谢产物的产生
this compound是花生四烯酸被脂氧合酶氧化后的产物 . 这些酶产生生物活性氧化代谢产物,包括白三烯、脂氧素和前列腺素,它们调节体内的炎症反应 .
生物技术生产
表达小鼠花生四烯酸8S-脂氧合酶的重组大肠杆菌细胞已被用于定量生物技术生产this compound . 这代表了一种生产这种化合物的新方法,可能在研究和医学领域有各种应用 .
6. 在细胞分裂和分化中的作用 this compound刺激过氧化物酶体增殖物激活受体(PPAR)-α,从而激活鼠角质形成细胞的细胞分裂和分化 . 这表明this compound可能在调节细胞生长和发育中发挥作用。
作用机制
Target of Action
8S-HETE, a product of the 8S-lipoxygenase (8S-LOX) enzyme, primarily targets suprabasal keratinocytes . The 8S-LOX enzyme is expressed in these cells and plays a significant role in epidermal tumor development in papillomas and induces chromosomal alterations in basal keratinocytes .
Mode of Action
This compound interacts with its targets, the suprabasal keratinocytes, by activating the protein kinase C (PKC) and peroxisome proliferator-activated receptor α (PPARα) . This activation leads to changes in the cells, such as increased differentiation and keratin-1 expression .
Biochemical Pathways
The 8S-LOX enzyme metabolizes arachidonic acid to produce this compound . This compound then stimulates the PPARα, thereby activating cell division and differentiation in murine keratinocytes . This process affects the lipoxygenase pathway, which is involved in the production of biologically active oxidative metabolites, including leukotrienes, lipoxins, and prostaglandins .
Result of Action
The action of this compound results in increased differentiation of keratinocytes and higher levels of keratin-1 expression . This suggests that this compound plays an important role in skin health and could potentially be involved in the pathogenesis of skin disorders .
Action Environment
The action of this compound is influenced by the environment within the cells and the overall physiological state of the organism. For instance, the expression of 8S-LOX, and thus the production of this compound, can be induced by irritation of the epidermis . Furthermore, the activity of this compound may be affected by other factors in the cellular environment, such as the presence of other signaling molecules and the overall health and state of the cells.
生化分析
Biochemical Properties
8S-HETE interacts with various enzymes, proteins, and other biomolecules. It activates mouse keratinocyte protein kinase C with an IC 50 of 100 µM . This compound also activates PPARα selectively at concentrations as low as 0.3 µM . The stereochemical assignment of the (S) enantiomer is based on comparison of chiral HPLC retention times to published results .
Cellular Effects
This compound has significant effects on various types of cells and cellular processes. It induces cellular hypertrophy in RL-14 cells through MAPK- and NF-κB-dependent mechanism . It also plays an important role in keratinocyte differentiation and that at least some of its effects are mediated by PPARα .
Molecular Mechanism
This compound exerts its effects at the molecular level through various mechanisms. It induces the NF-κB activity as well as it significantly induces the phosphorylation of ERK1/2 . Blocking the induction of NF-κB, ERK1/2, and sEH signaling pathways significantly inhibited this compound-induced cellular hypertrophy .
Temporal Effects in Laboratory Settings
The effects of this compound change over time in laboratory settings. Under the optimal hydroxylation conditions of pH 7.5, 30 C, 5% (v/v) ethanol, 15 g cells l-1, and 5 mM substrate, AA, EPA, and DHA were hydroxylated into 4.37 mM this compound, 3.77 mM 8S-HEPE, and 3.13 mM 10S-HDoHE for 60, 90, and 60 min, with 87, 75, and 63% molar conversions, respectively .
Metabolic Pathways
This compound is involved in various metabolic pathways. It is a major lipoxygenase product in PMA-treated murine epidermis . It is known to be metabolized by cyclooxygenases, lipoxygenases (LOXs), and cytochrome P450 (CYP) enzymes to form biologically active eicosanoids .
属性
IUPAC Name |
(5Z,8S,9E,11Z,14Z)-8-hydroxyicosa-5,9,11,14-tetraenoic acid | |
---|---|---|
Source | PubChem | |
URL | https://pubchem.ncbi.nlm.nih.gov | |
Description | Data deposited in or computed by PubChem | |
InChI |
InChI=1S/C20H32O3/c1-2-3-4-5-6-7-8-9-10-13-16-19(21)17-14-11-12-15-18-20(22)23/h6-7,9-11,13-14,16,19,21H,2-5,8,12,15,17-18H2,1H3,(H,22,23)/b7-6-,10-9-,14-11-,16-13+/t19-/m1/s1 | |
Source | PubChem | |
URL | https://pubchem.ncbi.nlm.nih.gov | |
Description | Data deposited in or computed by PubChem | |
InChI Key |
NLUNAYAEIJYXRB-VYOQERLCSA-N | |
Source | PubChem | |
URL | https://pubchem.ncbi.nlm.nih.gov | |
Description | Data deposited in or computed by PubChem | |
Canonical SMILES |
CCCCCC=CCC=CC=CC(CC=CCCCC(=O)O)O | |
Source | PubChem | |
URL | https://pubchem.ncbi.nlm.nih.gov | |
Description | Data deposited in or computed by PubChem | |
Isomeric SMILES |
CCCCC/C=C\C/C=C\C=C\[C@H](C/C=C\CCCC(=O)O)O | |
Source | PubChem | |
URL | https://pubchem.ncbi.nlm.nih.gov | |
Description | Data deposited in or computed by PubChem | |
Molecular Formula |
C20H32O3 | |
Source | PubChem | |
URL | https://pubchem.ncbi.nlm.nih.gov | |
Description | Data deposited in or computed by PubChem | |
DSSTOX Substance ID |
DTXSID101033819 | |
Record name | 8(S)-Hydroxyeicosatetraenoic acid | |
Source | EPA DSSTox | |
URL | https://comptox.epa.gov/dashboard/DTXSID101033819 | |
Description | DSSTox provides a high quality public chemistry resource for supporting improved predictive toxicology. | |
Molecular Weight |
320.5 g/mol | |
Source | PubChem | |
URL | https://pubchem.ncbi.nlm.nih.gov | |
Description | Data deposited in or computed by PubChem | |
Physical Description |
Solid | |
Record name | 8-HETE | |
Source | Human Metabolome Database (HMDB) | |
URL | http://www.hmdb.ca/metabolites/HMDB0004679 | |
Description | The Human Metabolome Database (HMDB) is a freely available electronic database containing detailed information about small molecule metabolites found in the human body. | |
Explanation | HMDB is offered to the public as a freely available resource. Use and re-distribution of the data, in whole or in part, for commercial purposes requires explicit permission of the authors and explicit acknowledgment of the source material (HMDB) and the original publication (see the HMDB citing page). We ask that users who download significant portions of the database cite the HMDB paper in any resulting publications. | |
CAS No. |
98462-03-4 | |
Record name | 8(S)-Hydroxyeicosatetraenoic acid | |
Source | CAS Common Chemistry | |
URL | https://commonchemistry.cas.org/detail?cas_rn=98462-03-4 | |
Description | CAS Common Chemistry is an open community resource for accessing chemical information. Nearly 500,000 chemical substances from CAS REGISTRY cover areas of community interest, including common and frequently regulated chemicals, and those relevant to high school and undergraduate chemistry classes. This chemical information, curated by our expert scientists, is provided in alignment with our mission as a division of the American Chemical Society. | |
Explanation | The data from CAS Common Chemistry is provided under a CC-BY-NC 4.0 license, unless otherwise stated. | |
Record name | 8(S)-Hydroxyeicosatetraenoic acid | |
Source | EPA DSSTox | |
URL | https://comptox.epa.gov/dashboard/DTXSID101033819 | |
Description | DSSTox provides a high quality public chemistry resource for supporting improved predictive toxicology. | |
Record name | 8(S)-Hydroxy-(5Z,9E,11Z,14Z)-eicosatetraenoic acid | |
Source | European Chemicals Agency (ECHA) | |
URL | https://echa.europa.eu/information-on-chemicals | |
Description | The European Chemicals Agency (ECHA) is an agency of the European Union which is the driving force among regulatory authorities in implementing the EU's groundbreaking chemicals legislation for the benefit of human health and the environment as well as for innovation and competitiveness. | |
Explanation | Use of the information, documents and data from the ECHA website is subject to the terms and conditions of this Legal Notice, and subject to other binding limitations provided for under applicable law, the information, documents and data made available on the ECHA website may be reproduced, distributed and/or used, totally or in part, for non-commercial purposes provided that ECHA is acknowledged as the source: "Source: European Chemicals Agency, http://echa.europa.eu/". Such acknowledgement must be included in each copy of the material. ECHA permits and encourages organisations and individuals to create links to the ECHA website under the following cumulative conditions: Links can only be made to webpages that provide a link to the Legal Notice page. | |
Record name | 8-HETE | |
Source | Human Metabolome Database (HMDB) | |
URL | http://www.hmdb.ca/metabolites/HMDB0004679 | |
Description | The Human Metabolome Database (HMDB) is a freely available electronic database containing detailed information about small molecule metabolites found in the human body. | |
Explanation | HMDB is offered to the public as a freely available resource. Use and re-distribution of the data, in whole or in part, for commercial purposes requires explicit permission of the authors and explicit acknowledgment of the source material (HMDB) and the original publication (see the HMDB citing page). We ask that users who download significant portions of the database cite the HMDB paper in any resulting publications. | |
Retrosynthesis Analysis
AI-Powered Synthesis Planning: Our tool employs the Template_relevance Pistachio, Template_relevance Bkms_metabolic, Template_relevance Pistachio_ringbreaker, Template_relevance Reaxys, Template_relevance Reaxys_biocatalysis model, leveraging a vast database of chemical reactions to predict feasible synthetic routes.
One-Step Synthesis Focus: Specifically designed for one-step synthesis, it provides concise and direct routes for your target compounds, streamlining the synthesis process.
Accurate Predictions: Utilizing the extensive PISTACHIO, BKMS_METABOLIC, PISTACHIO_RINGBREAKER, REAXYS, REAXYS_BIOCATALYSIS database, our tool offers high-accuracy predictions, reflecting the latest in chemical research and data.
Strategy Settings
Precursor scoring | Relevance Heuristic |
---|---|
Min. plausibility | 0.01 |
Model | Template_relevance |
Template Set | Pistachio/Bkms_metabolic/Pistachio_ringbreaker/Reaxys/Reaxys_biocatalysis |
Top-N result to add to graph | 6 |
Feasible Synthetic Routes
Q1: What is the primary mechanism of action for 8S-HETE in the body?
A1: this compound has been identified as a natural ligand for Peroxisome Proliferator-Activated Receptor alpha (PPARα). [, ] This interaction with PPARα appears to be key to its biological activity, particularly in inducing differentiation of specific cell types.
Q2: How does this compound contribute to keratinocyte differentiation?
A2: Studies using transgenic mice overexpressing 8-lipoxygenase (8-LOX), the enzyme responsible for this compound synthesis, revealed enhanced differentiation in skin, tongue, and stomach tissues. [] This differentiation was linked to increased keratin-1 expression, a marker of keratinocyte differentiation. Further experiments indicated that this compound's activation of PPARα plays a crucial role in this process. []
Q3: Are there other biological processes where this compound and PPARα are implicated?
A3: Research suggests that this compound, via PPARα activation, might be involved in inflammatory skin conditions. While the specific mechanisms are still under investigation, studies comparing 8-LOX activity in mice and humans suggest potentially analogous roles in inflammatory dermatoses. []
Q4: Beyond PPARα, does this compound interact with other targets?
A4: While PPARα is identified as a primary target, research suggests that this compound might possess alternative mechanisms of action. For instance, it can be further metabolized by enzymes like allene oxide synthase (cAOS) into various metabolites, including epoxides and other hydroxylated forms. [] These metabolites might have their own biological targets and effects, contributing to the overall influence of this compound.
Q5: What is the chemical structure of this compound?
A5: this compound is a monohydroxylated derivative of arachidonic acid. Its full chemical name is 8S-Hydroxy-5Z,8Z,11Z,14Z-eicosatetraenoic acid. While the provided research papers do not explicitly state its molecular formula and weight, these can be deduced from its structure. Spectroscopic data is not directly provided in the research abstracts.
Q6: Can this compound be produced using biotechnological approaches?
A6: Yes, recombinant Escherichia coli cells expressing mouse arachidonate 8S-lipoxygenase have been successfully used to produce this compound from arachidonic acid with high molar conversion rates. [, ] This approach highlights the potential for biotechnological production of this compound for research and potentially therapeutic applications.
Q7: Does modifying the structure of this compound affect its activity?
A7: While specific structure-activity relationship (SAR) studies for this compound were not detailed in the abstracts, research on related molecules like epoxyalcohols and trioxilins, derived from the same metabolic pathway, suggests that structural modifications can significantly influence PPARα activation. [] Further research focused on this compound analogs and their interaction with PPARα would be valuable to understand structural determinants of activity.
Q8: Are there specific analytical techniques used to study this compound?
A8: While not extensively detailed, the research papers mention techniques such as high-performance liquid chromatography (HPLC) for separating and identifying this compound and its metabolites. [, ] Other analytical methods commonly used in lipid analysis, like mass spectrometry and nuclear magnetic resonance spectroscopy, are likely employed for further characterization and quantification.
体外研究产品的免责声明和信息
请注意,BenchChem 上展示的所有文章和产品信息仅供信息参考。 BenchChem 上可购买的产品专为体外研究设计,这些研究在生物体外进行。体外研究,源自拉丁语 "in glass",涉及在受控实验室环境中使用细胞或组织进行的实验。重要的是要注意,这些产品没有被归类为药物或药品,他们没有得到 FDA 的批准,用于预防、治疗或治愈任何医疗状况、疾病或疾病。我们必须强调,将这些产品以任何形式引入人类或动物的身体都是法律严格禁止的。遵守这些指南对确保研究和实验的法律和道德标准的符合性至关重要。