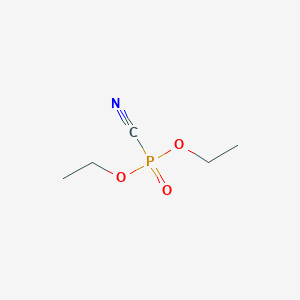
Diethyl cyanophosphonate
概述
描述
Diethyl cyanophosphonate (DEPC), with the chemical formula C₅H₁₀NO₃P and molecular weight 163.11 g/mol (CAS: 2942-58-7), is a phosphorus-based organochemical compound characterized by a cyano group (-CN) and two ethoxy groups (-OCH₂CH₃) attached to a central phosphorus atom . It is widely employed in organic synthesis as a coupling agent for peptide bond formation , nucleophilic substitution reactions (e.g., converting N-oxides to carbonitriles) , and fluorescence-based detection of nerve agent simulants . DEPC’s reactivity stems from its electrophilic phosphorus center and the cyano group’s strong electron-withdrawing nature, enabling selective transformations under mild conditions. However, it is highly toxic (T+), corrosive, and requires careful handling due to risks of decomposition .
准备方法
Diethyl cyanophosphonate can be synthesized by reacting diethyl phosphite with cyanogen bromide. The reaction mixture gradually heats up, and the product is obtained by vacuum distillation, collecting the fraction at 104-105°C under reduced pressure (2.53 kPa). The yield of this reaction is approximately 65% .
化学反应分析
Diethyl cyanophosphonate undergoes various chemical reactions, including:
Oxidation and Reduction:
Substitution Reactions: It is commonly used as a coupling reagent in peptide synthesis and as a reagent for the phosphorylation of phenols It also acts as an activating agent for the fluorescent derivatization of carboxylic acids, allowing separation by high-performance liquid chromatography.
Major Products: The major products formed from these reactions include phosphorylated phenols and fluorescent derivatives of carboxylic acids.
科学研究应用
Nerve Agent Simulant
Detection and Analysis
DCNP is recognized as a simulant for nerve agents, particularly Tabun. Its detection has been explored using various methods, including fluorescence-based sensors. For instance, a reversible fluorescent sensor utilizing fluorescein has been developed to detect DCNP effectively, demonstrating its potential for environmental monitoring and safety applications related to chemical warfare agents .
Research Studies
A study highlighted the hydrolysis of DCNP in the presence of amine-containing derivatives, showcasing its utility in developing remediation strategies for nerve agents. The research indicates that specific organocatalysts can facilitate the degradation of DCNP, thus contributing to methods for mitigating the effects of chemical warfare agents .
Organic Synthesis
Synthetic Intermediate
DCNP serves as an important intermediate in organic synthesis. It is utilized in the preparation of various phosphonate derivatives, which are crucial in the development of pharmaceuticals and agrochemicals. The compound's reactivity allows for the introduction of phosphonate groups into organic molecules, enhancing their biological activity .
Case Studies
In one case study, researchers employed DCNP in the synthesis of anti-inflammatory compounds. The phosphonate moiety was integral to the biological activity of these compounds, showcasing how DCNP can be leveraged to create therapeutically relevant molecules . Additionally, it has been used in click chemistry reactions for the detection of organophosphate pesticides, demonstrating its versatility in analytical applications .
Therapeutic Potential
Anti-inflammatory Applications
Recent patents have indicated that phosphonate compounds derived from DCNP exhibit anti-inflammatory properties. These compounds could potentially be developed into therapeutic agents for treating inflammatory diseases . The ability to modify DCNP to enhance its biological activity opens avenues for drug development.
Mechanistic Studies
Research has also focused on understanding the mechanisms by which DCNP and its derivatives exert anti-inflammatory effects. Studies suggest that these compounds may influence cellular pathways involved in inflammation, providing insights into their potential as therapeutic agents .
Summary Table of Applications
Application Area | Details |
---|---|
Nerve Agent Simulant | Used for detection via fluorescence sensors; aids in remediation strategies for nerve agents. |
Organic Synthesis | Acts as an intermediate for synthesizing phosphonate derivatives; enhances biological activity. |
Therapeutic Potential | Potential anti-inflammatory applications; modification leads to biologically active compounds. |
作用机制
Diethyl cyanophosphonate acts as a nerve agent simulant by inhibiting acetylcholinesterase, an enzyme responsible for breaking down the neurotransmitter acetylcholine. This inhibition leads to an accumulation of acetylcholine in the synaptic cleft, causing continuous stimulation of muscles, respiratory paralysis, and potentially death . The compound forms a stable complex with the enzyme, preventing its normal function .
相似化合物的比较
Diethyl Cyanophosphonate vs. Diethyl Chlorophosphate
- Structural Differences : DEPC contains a -CN group, while diethyl chlorophosphate has a -Cl substituent.
- Reactivity: DEPC’s cyano group facilitates cyclization reactions for fluorescence-based detection of nerve agents, whereas diethyl chlorophosphate lacks this capability . The -CN group also enhances DEPC’s nucleophilicity in substitution reactions compared to the less reactive -Cl group .
- Applications : Both are used as nerve agent simulants, but DEPC is uniquely applied in fluorogenic probes for selective Hg²⁺ and F⁻ detection .
This compound vs. Diethyl (2-Cyanoethyl)phosphonate
- Structural Differences : The latter features a -CH₂CH₂CN group instead of a direct -CN on phosphorus.
- Applications: Diethyl (2-cyanoethyl)phosphonate is used as an anti-inflammatory pharmaceutical agent, while DEPC serves as a synthetic reagent .
- Synthesis: Diethyl (2-cyanoethyl)phosphonate is synthesized via Arbuzov reactions, whereas DEPC is typically prepared through phosphorylation of cyanide sources .
This compound vs. Dimethyl Methylphosphonate (DMMP)
- Reactivity : DMMP (a methyl ester) is less reactive in hydrolysis due to its -OCH₃ groups, while DEPC’s -CN and -OCH₂CH₃ groups enable faster catalytic decomposition by polyoxoniobates (PONbs) .
- Safety : Both are used as chemical warfare simulants, but DEPC poses higher toxicity risks due to cyanide release .
This compound vs. Diethyl Trichloromethylphosphonate
- Functional Groups : The latter contains a -CCl₃ group, which is highly electrophilic but less nucleophilic than DEPC’s -CN.
- Applications : Diethyl trichloromethylphosphonate is used in specialty syntheses, whereas DEPC has broader utility in peptide coupling and biosensing .
Data Table: Comparative Analysis of this compound and Analogues
Key Research Findings
Fluorogenic Probes : DEPC’s cyclization with coumarin derivatives enables selective detection of nerve agents (e.g., DCNP) via fluorescence enhancement, a mechanism absent in diethyl chlorophosphate .
Catalytic Decomposition : PONbs catalyze DEPC hydrolysis more efficiently than DMMP due to the -CN group’s electronic effects .
Synthetic Utility: DEPC’s role in coupling Boc-Dap and 2-aminoquinoline highlights its superiority over other phosphonates in forming stable peptide bonds .
生物活性
Diethyl cyanophosphonate (DCNP) is an organophosphorus compound that has garnered interest due to its potential applications in biological systems, particularly as a nerve agent simulant and in synthetic organic chemistry. This article explores the biological activity of DCNP, focusing on its synthesis, mechanisms of action, and relevant case studies.
Chemical Structure and Properties
- Chemical Formula : CHNOP
- CAS Number : 2942-58-7
- Molecular Weight : 175.10 g/mol
DCNP is characterized by the presence of a cyanophosphonate group, which contributes to its reactivity and biological interactions. Its structure allows it to mimic certain nerve agents, making it a useful compound in research related to chemical warfare agents.
Synthesis of this compound
DCNP can be synthesized through various methods, including the reaction of phosphorus oxychloride with diethyl malonate and subsequent hydrolysis. The synthesis typically involves the following steps:
- Formation of the Phosphonyl Group : Reacting phosphorus oxychloride with diethyl malonate.
- Cyanation : Introducing a cyanide group to form the cyanophosphonate.
- Purification : Isolating the product through distillation or crystallization.
DCNP exhibits biological activity primarily through its interaction with cholinergic systems. As a nerve agent simulant, it inhibits acetylcholinesterase (AChE), leading to an accumulation of acetylcholine at synapses. This mechanism underlies its potential toxicity and therapeutic implications in treating nerve agent poisoning.
In Vitro Studies
In vitro studies have demonstrated that DCNP can affect various cellular processes:
- Cytotoxicity : Research has shown that DCNP exhibits cytotoxic effects on different cell lines. For instance, in studies involving CCRF-CEM leukemia cells, compounds derived from DCNP showed varying degrees of activity, with some derivatives being completely inactive (IC > 20 μg/mL) while others exhibited moderate activity (IC = 6.7 μg/mL) .
- Antifolate Activity : In synthetic pathways involving DCNP, researchers have explored its use in creating antifolate compounds. However, many synthesized derivatives were found to lack significant biological activity against cancer cell lines .
1. Nerve Agent Simulant Research
DCNP has been utilized as a model compound for studying the effects of nerve agents on biological systems. It serves as a safer alternative for evaluating antidotes and protective measures against actual chemical warfare agents. Studies have indicated that treatments with specific antidotes can mitigate the toxic effects induced by DCNP .
2. Decontamination Studies
In decontamination research involving simulated chemical agents, DCNP was used to evaluate the efficacy of various protective coatings and decontaminants. The protective ratios calculated from these studies help determine the effectiveness of treatments in preventing toxicity from exposure .
Summary of Findings
常见问题
Basic Research Questions
Q. What are the critical physico-chemical properties of DCNP relevant to experimental design?
DCNP (C₅H₁₀NO₃P) has a molecular weight of 163.11 g/mol, a density of 1.075 g/mL at 25°C, and a boiling point of 104–105°C under 19 mm Hg. Its stability in polar aprotic solvents (e.g., DMF, THF) makes it suitable for phosphorylation reactions. Researchers should prioritize inert atmosphere conditions (e.g., N₂) due to its sensitivity to moisture and potential HCN release during reactions .
Q. How is DCNP utilized as a coupling agent in peptide synthesis?
DCNP activates carboxyl groups for amide bond formation via in situ generation of reactive intermediates. For example, in peptide coupling:
- Dissolve the carboxyl substrate and amine in DMF at 0°C.
- Add DCNP (1.2 equiv) and a tertiary base (e.g., DIPEA, 3.0 equiv) to promote activation.
- Monitor reaction progress via LCMS to optimize yield and minimize side products like cyanate byproducts .
Q. What methodologies are effective for detecting DCNP in aqueous solutions?
Fluorescence-based detection using CdSe/ZnS quantum dots (QDs) and Au nanoparticles (AuNPs) leverages Förster Resonance Energy Transfer (FRET). DCNP disrupts QD-AuNP assemblies, restoring fluorescence quenched by FRET. Key steps:
- Prepare QD-AuNP composites via self-assembly.
- Calibrate fluorescence recovery against DCNP concentration (detection limit: ~10 nM).
- Validate specificity against interferents (e.g., diethyl chlorophosphate) using competitive assays .
Advanced Research Questions
Q. How can solvent-free synthesis of cyanohydrin O-phosphates using DCNP be optimized?
DCNP acts as both a phosphorylating agent and HCN source in solvent-free cyanohydrin O-phosphate synthesis:
- Mix aldehydes/ketones with DCNP (1.5 equiv) under mechanical stirring.
- Reaction completion (<30 min) is confirmed by TLC or NMR.
- Key factors: stoichiometric excess of DCNP, trace HCN catalysis, and temperature control (25–40°C) to prevent decomposition .
Q. What strategies improve selectivity in fluorescent probe design for DCNP detection?
Cyclization-induced fluorescence enhancement (CIEE) probes (e.g., coumarin derivatives) exploit DCNP’s nucleophilicity:
- Design probes with a latent fluorophore (e.g., 7-diethylamino coumarin) and a DCNP-reactive group (e.g., hydroxyl).
- DCNP triggers cyclization, restoring fluorescence (λₑₓ/λₑₘ = 350/450 nm).
- Validate selectivity using fluorogenic assays with nerve agent mimics (e.g., diethyl chlorophosphate) .
Q. How to resolve contradictions in proposed reaction mechanisms involving DCNP?
Conflicting mechanistic data (e.g., HCN participation in solvent-free synthesis) can be addressed by:
- Isotopic labeling (e.g., ¹³C-HCN) to track incorporation into products via NMR.
- Kinetic studies under varying HCN concentrations to establish rate dependencies.
- Computational modeling (DFT) to compare activation energies of proposed pathways .
Q. What methodologies are recommended for toxicological profiling of DCNP?
- In vitro assays : Measure acetylcholinesterase (AChE) inhibition using Ellman’s method (IC₅₀ determination).
- In silico modeling : Use QSAR models for organophosphates to predict acute toxicity (e.g., LD₅₀).
- Class-based extrapolation : Compare DCNP’s reactivity with structurally related nerve agents (e.g., sarin analogs) .
Q. How to analyze contradictory data in FRET-based DCNP detection systems?
Discrepancies in fluorescence recovery efficiency may arise from:
- QD-AuNP spacing : Optimize self-assembly conditions (e.g., pH, ionic strength) to ensure consistent FRET efficiency.
- Matrix effects : Perform spike-and-recovery experiments in complex matrices (e.g., serum) to assess interference.
- Data normalization : Use internal standards (e.g., rhodamine B) to correct for instrumental variability .
属性
IUPAC Name |
diethoxyphosphorylformonitrile | |
---|---|---|
Source | PubChem | |
URL | https://pubchem.ncbi.nlm.nih.gov | |
Description | Data deposited in or computed by PubChem | |
InChI |
InChI=1S/C5H10NO3P/c1-3-8-10(7,5-6)9-4-2/h3-4H2,1-2H3 | |
Source | PubChem | |
URL | https://pubchem.ncbi.nlm.nih.gov | |
Description | Data deposited in or computed by PubChem | |
InChI Key |
ZWWWLCMDTZFSOO-UHFFFAOYSA-N | |
Source | PubChem | |
URL | https://pubchem.ncbi.nlm.nih.gov | |
Description | Data deposited in or computed by PubChem | |
Canonical SMILES |
CCOP(=O)(C#N)OCC | |
Source | PubChem | |
URL | https://pubchem.ncbi.nlm.nih.gov | |
Description | Data deposited in or computed by PubChem | |
Molecular Formula |
C5H10NO3P | |
Source | PubChem | |
URL | https://pubchem.ncbi.nlm.nih.gov | |
Description | Data deposited in or computed by PubChem | |
DSSTOX Substance ID |
DTXSID90183639 | |
Record name | Diethylphosphorocyanidate | |
Source | EPA DSSTox | |
URL | https://comptox.epa.gov/dashboard/DTXSID90183639 | |
Description | DSSTox provides a high quality public chemistry resource for supporting improved predictive toxicology. | |
Molecular Weight |
163.11 g/mol | |
Source | PubChem | |
URL | https://pubchem.ncbi.nlm.nih.gov | |
Description | Data deposited in or computed by PubChem | |
Physical Description |
Yellow liquid; [Alfa Aesar MSDS] | |
Record name | Diethyl cyanophosphonate | |
Source | Haz-Map, Information on Hazardous Chemicals and Occupational Diseases | |
URL | https://haz-map.com/Agents/20583 | |
Description | Haz-Map® is an occupational health database designed for health and safety professionals and for consumers seeking information about the adverse effects of workplace exposures to chemical and biological agents. | |
Explanation | Copyright (c) 2022 Haz-Map(R). All rights reserved. Unless otherwise indicated, all materials from Haz-Map are copyrighted by Haz-Map(R). No part of these materials, either text or image may be used for any purpose other than for personal use. Therefore, reproduction, modification, storage in a retrieval system or retransmission, in any form or by any means, electronic, mechanical or otherwise, for reasons other than personal use, is strictly prohibited without prior written permission. | |
CAS No. |
2942-58-7 | |
Record name | Diethyl cyanophosphonate | |
Source | CAS Common Chemistry | |
URL | https://commonchemistry.cas.org/detail?cas_rn=2942-58-7 | |
Description | CAS Common Chemistry is an open community resource for accessing chemical information. Nearly 500,000 chemical substances from CAS REGISTRY cover areas of community interest, including common and frequently regulated chemicals, and those relevant to high school and undergraduate chemistry classes. This chemical information, curated by our expert scientists, is provided in alignment with our mission as a division of the American Chemical Society. | |
Explanation | The data from CAS Common Chemistry is provided under a CC-BY-NC 4.0 license, unless otherwise stated. | |
Record name | Diethylphosphorocyanidate | |
Source | ChemIDplus | |
URL | https://pubchem.ncbi.nlm.nih.gov/substance/?source=chemidplus&sourceid=0002942587 | |
Description | ChemIDplus is a free, web search system that provides access to the structure and nomenclature authority files used for the identification of chemical substances cited in National Library of Medicine (NLM) databases, including the TOXNET system. | |
Record name | Diethylphosphorocyanidate | |
Source | EPA DSSTox | |
URL | https://comptox.epa.gov/dashboard/DTXSID90183639 | |
Description | DSSTox provides a high quality public chemistry resource for supporting improved predictive toxicology. | |
Record name | Diethyl cyanidophosphate | |
Source | European Chemicals Agency (ECHA) | |
URL | https://echa.europa.eu/substance-information/-/substanceinfo/100.019.033 | |
Description | The European Chemicals Agency (ECHA) is an agency of the European Union which is the driving force among regulatory authorities in implementing the EU's groundbreaking chemicals legislation for the benefit of human health and the environment as well as for innovation and competitiveness. | |
Explanation | Use of the information, documents and data from the ECHA website is subject to the terms and conditions of this Legal Notice, and subject to other binding limitations provided for under applicable law, the information, documents and data made available on the ECHA website may be reproduced, distributed and/or used, totally or in part, for non-commercial purposes provided that ECHA is acknowledged as the source: "Source: European Chemicals Agency, http://echa.europa.eu/". Such acknowledgement must be included in each copy of the material. ECHA permits and encourages organisations and individuals to create links to the ECHA website under the following cumulative conditions: Links can only be made to webpages that provide a link to the Legal Notice page. | |
Record name | DIETHYLPHOSPHOROCYANIDATE | |
Source | FDA Global Substance Registration System (GSRS) | |
URL | https://gsrs.ncats.nih.gov/ginas/app/beta/substances/PAD4WPZ7T0 | |
Description | The FDA Global Substance Registration System (GSRS) enables the efficient and accurate exchange of information on what substances are in regulated products. Instead of relying on names, which vary across regulatory domains, countries, and regions, the GSRS knowledge base makes it possible for substances to be defined by standardized, scientific descriptions. | |
Explanation | Unless otherwise noted, the contents of the FDA website (www.fda.gov), both text and graphics, are not copyrighted. They are in the public domain and may be republished, reprinted and otherwise used freely by anyone without the need to obtain permission from FDA. Credit to the U.S. Food and Drug Administration as the source is appreciated but not required. | |
Synthesis routes and methods
Procedure details
Retrosynthesis Analysis
AI-Powered Synthesis Planning: Our tool employs the Template_relevance Pistachio, Template_relevance Bkms_metabolic, Template_relevance Pistachio_ringbreaker, Template_relevance Reaxys, Template_relevance Reaxys_biocatalysis model, leveraging a vast database of chemical reactions to predict feasible synthetic routes.
One-Step Synthesis Focus: Specifically designed for one-step synthesis, it provides concise and direct routes for your target compounds, streamlining the synthesis process.
Accurate Predictions: Utilizing the extensive PISTACHIO, BKMS_METABOLIC, PISTACHIO_RINGBREAKER, REAXYS, REAXYS_BIOCATALYSIS database, our tool offers high-accuracy predictions, reflecting the latest in chemical research and data.
Strategy Settings
Precursor scoring | Relevance Heuristic |
---|---|
Min. plausibility | 0.01 |
Model | Template_relevance |
Template Set | Pistachio/Bkms_metabolic/Pistachio_ringbreaker/Reaxys/Reaxys_biocatalysis |
Top-N result to add to graph | 6 |
Feasible Synthetic Routes
体外研究产品的免责声明和信息
请注意,BenchChem 上展示的所有文章和产品信息仅供信息参考。 BenchChem 上可购买的产品专为体外研究设计,这些研究在生物体外进行。体外研究,源自拉丁语 "in glass",涉及在受控实验室环境中使用细胞或组织进行的实验。重要的是要注意,这些产品没有被归类为药物或药品,他们没有得到 FDA 的批准,用于预防、治疗或治愈任何医疗状况、疾病或疾病。我们必须强调,将这些产品以任何形式引入人类或动物的身体都是法律严格禁止的。遵守这些指南对确保研究和实验的法律和道德标准的符合性至关重要。