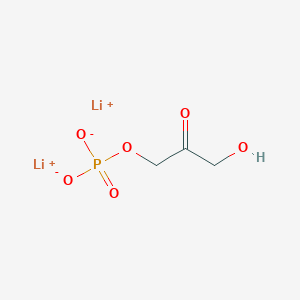
二羟基丙酮磷酸二锂盐
描述
Dihydroxyacetone phosphate dilithium salt is a chemical compound with the empirical formula C₃H₅Li₂O₆P. It is a metabolic intermediate involved in various biochemical pathways, including glycolysis, gluconeogenesis, glycerol metabolism, phosphatidic acid synthesis, fat metabolism, and the Calvin cycle .
科学研究应用
Dihydroxyacetone phosphate dilithium salt is widely used in scientific research due to its role as a metabolic intermediate. Its applications include:
作用机制
Target of Action
Dihydroxyacetone phosphate (DHAP) primarily targets enzymes such as fructose-bisphosphate aldolase (ALDOA) and triosephosphate isomerase . These enzymes play a crucial role in various metabolic pathways, including glycolysis and gluconeogenesis .
Mode of Action
DHAP interacts with its target enzymes by serving as a substrate. It is used in studies involving the metabolism of three-carbon sugar pools and their roles in physiological and physical processes . The interaction of DHAP with these enzymes leads to various biochemical reactions that are essential for energy production and other metabolic processes .
Biochemical Pathways
DHAP is a metabolic intermediate involved in many pathways, including glycolysis, gluconeogenesis, glycerol metabolism, phosphatidic acid synthesis, fat metabolism, and the Calvin cycle . In glycolysis, for instance, DHAP is converted to glyceraldehyde 3-phosphate, which then undergoes further reactions to produce ATP, the cell’s main energy currency .
Result of Action
The action of DHAP at the molecular and cellular levels results in the production of energy and the regulation of various metabolic processes. By serving as a substrate for key enzymes, DHAP facilitates the breakdown of glucose and the synthesis of other important molecules, contributing to the overall metabolic functioning of the cell .
生化分析
Biochemical Properties
Dihydroxyacetone phosphate dilithium salt plays a crucial role in biochemical reactions. It interacts with various enzymes, proteins, and other biomolecules. For instance, it may be used as a substrate to help identify, differentiate, and characterize fructose-bisphosphate aldolase (ALDOA) and triosephosphate isomerase . The nature of these interactions is essential for the metabolism of three-carbon sugar pools and their roles in physiological and physical processes .
Cellular Effects
Dihydroxyacetone phosphate dilithium salt has significant effects on various types of cells and cellular processes. It influences cell function, including any impact on cell signaling pathways, gene expression, and cellular metabolism . For example, it may be used to study cellular glycation stress .
Molecular Mechanism
The molecular mechanism of action of Dihydroxyacetone phosphate dilithium salt involves its effects at the molecular level, including any binding interactions with biomolecules, enzyme inhibition or activation, and changes in gene expression . It is rapidly and reversibly isomerised to glyceraldehyde 3-phosphate .
Temporal Effects in Laboratory Settings
The effects of Dihydroxyacetone phosphate dilithium salt change over time in laboratory settings. Information on the product’s stability, degradation, and any long-term effects on cellular function observed in in vitro or in vivo studies is crucial for understanding its biochemical properties .
Dosage Effects in Animal Models
The effects of Dihydroxyacetone phosphate dilithium salt vary with different dosages in animal models. This includes any threshold effects observed in these studies, as well as any toxic or adverse effects at high doses .
Metabolic Pathways
Dihydroxyacetone phosphate dilithium salt is involved in several metabolic pathways. It interacts with various enzymes or cofactors, and this interaction can affect metabolic flux or metabolite levels . For instance, it is involved in the Calvin cycle in plants and glycolysis .
Transport and Distribution
Dihydroxyacetone phosphate dilithium salt is transported and distributed within cells and tissues. It could interact with transporters or binding proteins, affecting its localization or accumulation .
Subcellular Localization
The subcellular localization of Dihydroxyacetone phosphate dilithium salt and any effects on its activity or function are crucial for understanding its biochemical properties. This could include any targeting signals or post-translational modifications that direct it to specific compartments or organelles .
准备方法
Synthetic Routes and Reaction Conditions
Dihydroxyacetone phosphate dilithium salt can be synthesized through both chemical and enzymatic routes. Chemical synthetic routes often focus on producing a storable precursor that can be easily converted to dihydroxyacetone phosphate immediately before its use . One such method involves the Lewis acid-mediated regioselective epoxide ring opening with dibenzyl phosphate, followed by catalytic oxidation of the secondary alcohol by tetrapropylammonium perruthenate/N-methylmorpholine N-oxide, leading to dibenzyl-3-benzylhydroxyacetone phosphate. This stable stock material is then quantitatively hydrogenolyzed into dihydroxyacetone phosphate .
Enzymatic routes typically involve integrating the formation of dihydroxyacetone phosphate with upstream or downstream catalytic steps, leading to multi-enzyme arrangements with up to seven enzymes operating simultaneously . These routes often start from cheap unphosphorylated precursors such as dihydroxyacetone or glycerol, which are then converted to dihydroxyacetone phosphate through a series of enzymatic reactions .
Industrial Production Methods
Industrial production of dihydroxyacetone phosphate dilithium salt often relies on enzymatic methods due to their mild reaction conditions and suitability for multifunctional complex molecules . These methods are scalable and can be integrated into existing biochemical production processes.
化学反应分析
Types of Reactions
Dihydroxyacetone phosphate dilithium salt undergoes various types of chemical reactions, including:
Oxidation: Catalytic oxidation of secondary alcohols.
Reduction: Reduction of ketone groups.
Substitution: Nucleophilic substitution reactions involving phosphate groups.
Common Reagents and Conditions
Common reagents used in these reactions include:
Oxidizing agents: Tetrapropylammonium perruthenate/N-methylmorpholine N-oxide.
Reducing agents: Sodium borohydride.
Nucleophiles: Dibenzyl phosphate.
Major Products
Major products formed from these reactions include various phosphorylated intermediates and derivatives of dihydroxyacetone phosphate .
相似化合物的比较
Similar Compounds
Dihydroxyacetone phosphate lithium salt: Similar in structure and function but differs in the cation present.
Dihydroxyacetone phosphate hemimagnesium salt hydrate: Another variant with magnesium as the cation.
Uniqueness
Dihydroxyacetone phosphate dilithium salt is unique due to its specific cation composition, which can influence its solubility and reactivity in biochemical reactions. This makes it particularly suitable for certain enzymatic processes and industrial applications .
属性
IUPAC Name |
dilithium;(3-hydroxy-2-oxopropyl) phosphate | |
---|---|---|
Source | PubChem | |
URL | https://pubchem.ncbi.nlm.nih.gov | |
Description | Data deposited in or computed by PubChem | |
InChI |
InChI=1S/C3H7O6P.2Li/c4-1-3(5)2-9-10(6,7)8;;/h4H,1-2H2,(H2,6,7,8);;/q;2*+1/p-2 | |
Source | PubChem | |
URL | https://pubchem.ncbi.nlm.nih.gov | |
Description | Data deposited in or computed by PubChem | |
InChI Key |
QWIKESRFRWLYIA-UHFFFAOYSA-L | |
Source | PubChem | |
URL | https://pubchem.ncbi.nlm.nih.gov | |
Description | Data deposited in or computed by PubChem | |
Canonical SMILES |
[Li+].[Li+].C(C(=O)COP(=O)([O-])[O-])O | |
Source | PubChem | |
URL | https://pubchem.ncbi.nlm.nih.gov | |
Description | Data deposited in or computed by PubChem | |
Molecular Formula |
C3H5Li2O6P | |
Source | PubChem | |
URL | https://pubchem.ncbi.nlm.nih.gov | |
Description | Data deposited in or computed by PubChem | |
DSSTOX Substance ID |
DTXSID10585136 | |
Record name | Dilithium 3-hydroxy-2-oxopropyl phosphate | |
Source | EPA DSSTox | |
URL | https://comptox.epa.gov/dashboard/DTXSID10585136 | |
Description | DSSTox provides a high quality public chemistry resource for supporting improved predictive toxicology. | |
Molecular Weight |
182.0 g/mol | |
Source | PubChem | |
URL | https://pubchem.ncbi.nlm.nih.gov | |
Description | Data deposited in or computed by PubChem | |
CAS No. |
102783-56-2 | |
Record name | Dilithium 3-hydroxy-2-oxopropyl phosphate | |
Source | EPA DSSTox | |
URL | https://comptox.epa.gov/dashboard/DTXSID10585136 | |
Description | DSSTox provides a high quality public chemistry resource for supporting improved predictive toxicology. | |
Retrosynthesis Analysis
AI-Powered Synthesis Planning: Our tool employs the Template_relevance Pistachio, Template_relevance Bkms_metabolic, Template_relevance Pistachio_ringbreaker, Template_relevance Reaxys, Template_relevance Reaxys_biocatalysis model, leveraging a vast database of chemical reactions to predict feasible synthetic routes.
One-Step Synthesis Focus: Specifically designed for one-step synthesis, it provides concise and direct routes for your target compounds, streamlining the synthesis process.
Accurate Predictions: Utilizing the extensive PISTACHIO, BKMS_METABOLIC, PISTACHIO_RINGBREAKER, REAXYS, REAXYS_BIOCATALYSIS database, our tool offers high-accuracy predictions, reflecting the latest in chemical research and data.
Strategy Settings
Precursor scoring | Relevance Heuristic |
---|---|
Min. plausibility | 0.01 |
Model | Template_relevance |
Template Set | Pistachio/Bkms_metabolic/Pistachio_ringbreaker/Reaxys/Reaxys_biocatalysis |
Top-N result to add to graph | 6 |
Feasible Synthetic Routes
体外研究产品的免责声明和信息
请注意,BenchChem 上展示的所有文章和产品信息仅供信息参考。 BenchChem 上可购买的产品专为体外研究设计,这些研究在生物体外进行。体外研究,源自拉丁语 "in glass",涉及在受控实验室环境中使用细胞或组织进行的实验。重要的是要注意,这些产品没有被归类为药物或药品,他们没有得到 FDA 的批准,用于预防、治疗或治愈任何医疗状况、疾病或疾病。我们必须强调,将这些产品以任何形式引入人类或动物的身体都是法律严格禁止的。遵守这些指南对确保研究和实验的法律和道德标准的符合性至关重要。