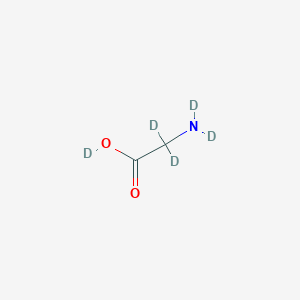
Glycine-d5
概述
描述
Glycine-d5 is an isotopically labeled compound of glycine, where all five hydrogen atoms are replaced by deuterium. This compound is primarily used as an internal standard in various analytical techniques, such as gas chromatography-mass spectrometry (GC-MS) and liquid chromatography-mass spectrometry (LC-MS), to quantify glycine levels in biological samples .
科学研究应用
Glycine-d5 is widely used in scientific research due to its isotopic labeling. Some key applications include:
Chemistry: Used as an internal standard in analytical techniques to quantify glycine levels.
Biology: Employed in metabolic studies to trace glycine pathways and understand its role in various biological processes.
Medicine: Used in pharmacokinetic studies to understand the metabolism and distribution of glycine in the body.
Industry: Applied in quality control processes to ensure the accuracy and precision of analytical measurements.
作用机制
Target of Action
Glycine-d5, a deuterium-labeled form of glycine, primarily targets the N-methyl-D-aspartic acid (NMDA) receptors and glycine receptors (GlyRs) . These receptors play crucial roles in the central nervous system. NMDA receptors are involved in excitatory neurotransmission , while GlyRs mediate inhibitory neurotransmission .
Mode of Action
This compound acts as an inhibitory neurotransmitter in the central nervous system (CNS) and also as a co-agonist along with glutamate, facilitating an excitatory potential at the glutaminergic NMDA receptors . In the CNS, there exist strychnine-sensitive glycine binding sites as well as strychnine-insensitive glycine binding sites. The strychnine-insensitive glycine-binding site is located on the NMDA receptor complex .
Biochemical Pathways
This compound is involved in several biochemical pathways. It is synthesized from serine, threonine, choline, and hydroxyproline via inter-organ metabolism primarily involving the liver and kidneys . Glycine degradation occurs through three pathways: the glycine cleavage system (GCS), serine hydroxymethyltransferase, and conversion to glyoxylate by peroxisomal d-amino acid oxidase . Among these pathways, GCS is the major enzyme initiating glycine degradation to form ammonia and CO2 .
Pharmacokinetics
The pharmacokinetics of glycine, and by extension this compound, suggest that it is transported into brain tissue by passive diffusion . This transportation is crucial for its role as a neurotransmitter in the CNS.
Result of Action
The action of this compound at its target receptors leads to various molecular and cellular effects. It has been shown to confer neuroprotection against neurodegeneration and memory impairment by regulating c-Jun N-terminal kinase in the mouse brain . Glycine also boosts satellite cell proliferation and muscle regeneration by increasing activation of mammalian target of rapamycin complex 1 (mTORC1) .
Action Environment
Environmental factors can influence the action, efficacy, and stability of this compound. Factors such as diet quality and quantity, physical activity, exposure to noise or fine dust, sleep patterns, smoking, stress, depression, and socioeconomic status can all potentially impact the effectiveness of this compound . .
生化分析
Biochemical Properties
Glycine-d5, like its non-deuterated counterpart, participates in various biochemical reactions. It interacts with enzymes, proteins, and other biomolecules. For instance, it plays a role as an allosteric regulator of NMDA receptors . The nature of these interactions involves the formation and breaking of chemical bonds, leading to various physiological effects.
Cellular Effects
This compound has significant effects on various types of cells and cellular processes. It influences cell function, including impacts on cell signaling pathways, gene expression, and cellular metabolism . For example, this compound has been shown to have neuroprotective effects against neuroapoptosis, neuroinflammation, synaptic dysfunction, and memory impairment .
Molecular Mechanism
The molecular mechanism of action of this compound involves its interactions at the molecular level. It exerts its effects through binding interactions with biomolecules, enzyme inhibition or activation, and changes in gene expression . For instance, it has been found to inhibit the upregulation of phospho-c-Jun N-terminal kinase (p-JNK) levels, which are typically elevated during oxidative stress-mediated neurodegeneration .
Temporal Effects in Laboratory Settings
The effects of this compound change over time in laboratory settings. It has been observed that this compound inhibits oxidative stress and significantly upregulates the expression of antioxidant proteins that had been suppressed in the mouse brain . These effects indicate the stability of this compound and its long-term effects on cellular function in in vitro or in vivo studies.
Dosage Effects in Animal Models
The effects of this compound vary with different dosages in animal models. For instance, in metabolic disorders associated with obesity, lower circulating glycine levels have been consistently observed, and clinical studies suggest beneficial effects induced by glycine supplementation . The specific threshold effects and any toxic or adverse effects at high doses of this compound remain to be further investigated.
Metabolic Pathways
This compound is involved in several metabolic pathways. It is synthesized from serine, threonine, choline, and hydroxyproline via inter-organ metabolism involving primarily the liver and kidneys . Glycine degradation occurs through three pathways: the glycine cleavage system (GCS), serine hydroxymethyltransferase, and conversion to glyoxylate by peroxisomal d-amino acid oxidase .
Transport and Distribution
This compound is transported and distributed within cells and tissues. It interacts with specific transporters or binding proteins, affecting its localization or accumulation . For instance, the glial glycine-selective transporter GlyT1 is the main regulator of synaptic glycine concentrations .
准备方法
Synthetic Routes and Reaction Conditions
The preparation of Glycine-d5 involves the deuteration of glycine. One common method is the exchange of hydrogen atoms in glycine with deuterium using deuterated water (D2O) under acidic or basic conditions. The reaction typically involves heating glycine in D2O with a catalyst to facilitate the exchange process .
Industrial Production Methods
Industrial production of this compound follows similar principles but on a larger scale. The process involves the use of deuterated reagents and solvents to ensure high isotopic purity. The reaction conditions are optimized to maximize the yield and purity of the final product. The product is then purified using techniques such as crystallization or chromatography .
化学反应分析
Types of Reactions
Glycine-d5 undergoes various chemical reactions similar to its non-deuterated counterpart. These include:
Oxidation: this compound can be oxidized to form glyoxylate-d5 and ammonia.
Reduction: It can be reduced to form aminoacetaldehyde-d5.
Substitution: This compound can undergo nucleophilic substitution reactions to form various derivatives.
Common Reagents and Conditions
Oxidation: Common oxidizing agents include potassium permanganate and hydrogen peroxide.
Reduction: Reducing agents such as sodium borohydride or lithium aluminum hydride are used.
Substitution: Nucleophiles like halides or amines are used under basic or acidic conditions.
Major Products
Oxidation: Glyoxylate-d5 and ammonia.
Reduction: Aminoacetaldehyde-d5.
Substitution: Various glycine derivatives depending on the nucleophile used.
相似化合物的比较
Similar Compounds
Glycine-2,2-d2: Another deuterated form of glycine with two deuterium atoms.
Glycine-13C2: A carbon-13 labeled glycine.
L-Alanine-2,3,3,3-d4: A deuterated form of alanine.
Uniqueness
Glycine-d5 is unique due to its complete deuteration, which provides a distinct mass shift (M+5) compared to other partially deuterated or carbon-labeled glycine compounds. This makes it particularly useful in studies requiring high isotopic purity and precise quantification .
属性
IUPAC Name |
deuterio 2,2-dideuterio-2-(dideuterioamino)acetate | |
---|---|---|
Source | PubChem | |
URL | https://pubchem.ncbi.nlm.nih.gov | |
Description | Data deposited in or computed by PubChem | |
InChI |
InChI=1S/C2H5NO2/c3-1-2(4)5/h1,3H2,(H,4,5)/i1D2/hD3 | |
Source | PubChem | |
URL | https://pubchem.ncbi.nlm.nih.gov | |
Description | Data deposited in or computed by PubChem | |
InChI Key |
DHMQDGOQFOQNFH-LGLHGEJLSA-N | |
Source | PubChem | |
URL | https://pubchem.ncbi.nlm.nih.gov | |
Description | Data deposited in or computed by PubChem | |
Canonical SMILES |
C(C(=O)O)N | |
Source | PubChem | |
URL | https://pubchem.ncbi.nlm.nih.gov | |
Description | Data deposited in or computed by PubChem | |
Isomeric SMILES |
[2H]C([2H])(C(=O)O[2H])N([2H])[2H] | |
Source | PubChem | |
URL | https://pubchem.ncbi.nlm.nih.gov | |
Description | Data deposited in or computed by PubChem | |
Molecular Formula |
C2H5NO2 | |
Source | PubChem | |
URL | https://pubchem.ncbi.nlm.nih.gov | |
Description | Data deposited in or computed by PubChem | |
Molecular Weight |
80.10 g/mol | |
Source | PubChem | |
URL | https://pubchem.ncbi.nlm.nih.gov | |
Description | Data deposited in or computed by PubChem | |
CAS No. |
4896-77-9 | |
Record name | Glycine-d5 | |
Source | CAS Common Chemistry | |
URL | https://commonchemistry.cas.org/detail?cas_rn=4896-77-9 | |
Description | CAS Common Chemistry is an open community resource for accessing chemical information. Nearly 500,000 chemical substances from CAS REGISTRY cover areas of community interest, including common and frequently regulated chemicals, and those relevant to high school and undergraduate chemistry classes. This chemical information, curated by our expert scientists, is provided in alignment with our mission as a division of the American Chemical Society. | |
Explanation | The data from CAS Common Chemistry is provided under a CC-BY-NC 4.0 license, unless otherwise stated. | |
Record name | Deuterated glycine | |
Source | ChemIDplus | |
URL | https://pubchem.ncbi.nlm.nih.gov/substance/?source=chemidplus&sourceid=0004896779 | |
Description | ChemIDplus is a free, web search system that provides access to the structure and nomenclature authority files used for the identification of chemical substances cited in National Library of Medicine (NLM) databases, including the TOXNET system. | |
Record name | (2H5)glycine | |
Source | European Chemicals Agency (ECHA) | |
URL | https://echa.europa.eu/substance-information/-/substanceinfo/100.023.198 | |
Description | The European Chemicals Agency (ECHA) is an agency of the European Union which is the driving force among regulatory authorities in implementing the EU's groundbreaking chemicals legislation for the benefit of human health and the environment as well as for innovation and competitiveness. | |
Explanation | Use of the information, documents and data from the ECHA website is subject to the terms and conditions of this Legal Notice, and subject to other binding limitations provided for under applicable law, the information, documents and data made available on the ECHA website may be reproduced, distributed and/or used, totally or in part, for non-commercial purposes provided that ECHA is acknowledged as the source: "Source: European Chemicals Agency, http://echa.europa.eu/". Such acknowledgement must be included in each copy of the material. ECHA permits and encourages organisations and individuals to create links to the ECHA website under the following cumulative conditions: Links can only be made to webpages that provide a link to the Legal Notice page. | |
Synthesis routes and methods I
Procedure details
Synthesis routes and methods II
Procedure details
Synthesis routes and methods III
Procedure details
Synthesis routes and methods IV
Procedure details
Retrosynthesis Analysis
AI-Powered Synthesis Planning: Our tool employs the Template_relevance Pistachio, Template_relevance Bkms_metabolic, Template_relevance Pistachio_ringbreaker, Template_relevance Reaxys, Template_relevance Reaxys_biocatalysis model, leveraging a vast database of chemical reactions to predict feasible synthetic routes.
One-Step Synthesis Focus: Specifically designed for one-step synthesis, it provides concise and direct routes for your target compounds, streamlining the synthesis process.
Accurate Predictions: Utilizing the extensive PISTACHIO, BKMS_METABOLIC, PISTACHIO_RINGBREAKER, REAXYS, REAXYS_BIOCATALYSIS database, our tool offers high-accuracy predictions, reflecting the latest in chemical research and data.
Strategy Settings
Precursor scoring | Relevance Heuristic |
---|---|
Min. plausibility | 0.01 |
Model | Template_relevance |
Template Set | Pistachio/Bkms_metabolic/Pistachio_ringbreaker/Reaxys/Reaxys_biocatalysis |
Top-N result to add to graph | 6 |
Feasible Synthetic Routes
Q1: What is the primary use of Glycine-d5 in scientific research?
A1: this compound is primarily used as a labeled compound in various research applications. Its deuterium atoms allow for easy tracking of the molecule and its fragments in complex mixtures using techniques like mass spectrometry. For example, researchers used this compound, along with other labeled glycine isotopes, to study the degradation pathways of glycine in simulated Martian conditions. [] They found that decarboxylation, leading to the loss of carbon dioxide, was the first step in glycine degradation, regardless of the presence of perchlorates. []
Q2: How does deuteration in this compound contribute to its utility in spin-trapping experiments?
A2: this compound, where all five hydrogen atoms are replaced with deuterium, provides valuable insights into the structure and reactions of radicals formed from glycine. [] When used in Electron Spin Resonance (ESR) spin-trapping experiments, the distinct hyperfine coupling constants of deuterium compared to hydrogen help to unambiguously identify the trapped radical species. [] This is particularly useful in studying complex reaction mixtures where multiple radical species might be present.
Q3: Can you provide an example of a chemical synthesis method where this compound is utilized?
A3: this compound serves as a valuable precursor in organic synthesis. One specific application is the synthesis of Glycolic-d2 acid (HOCD2CO2H). [] In this process, researchers employed two methods: the Cannizzaro rearrangement of glyoxal-d2 and the reaction of nitrous acid with this compound. [] This highlights the versatility of this compound as a starting material for preparing other deuterated compounds.
Q4: Are there any studies investigating the impact of deuteration on the physical properties of Glycine?
A4: Yes, research has explored how deuteration affects the nuclear spin lattice relaxation of glycine. [] Specifically, studies on this compound in solid-state demonstrated that slow sample rotation could enhance the spin-lattice relaxation of rigid sites when neighboring fast-relaxing sites are present. [] This finding has implications for understanding molecular dynamics and interactions within solid-state samples.
体外研究产品的免责声明和信息
请注意,BenchChem 上展示的所有文章和产品信息仅供信息参考。 BenchChem 上可购买的产品专为体外研究设计,这些研究在生物体外进行。体外研究,源自拉丁语 "in glass",涉及在受控实验室环境中使用细胞或组织进行的实验。重要的是要注意,这些产品没有被归类为药物或药品,他们没有得到 FDA 的批准,用于预防、治疗或治愈任何医疗状况、疾病或疾病。我们必须强调,将这些产品以任何形式引入人类或动物的身体都是法律严格禁止的。遵守这些指南对确保研究和实验的法律和道德标准的符合性至关重要。