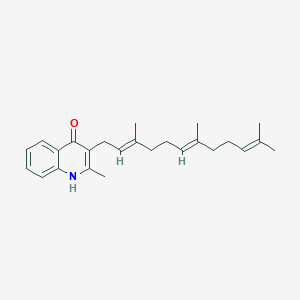
奥拉霉素D
描述
Aurachin D is a farnesylated quinolone alkaloid naturally produced by myxobacteria of the genus Stigmatella and some actinobacterial strains . It is a potent inhibitor of cytochrome bd oxidases, which are potential targets in the treatment of infectious diseases . Aurachin D has garnered significant interest due to its remarkable activities against various pathogens, including Leishmania donovani, the causative agent of leishmaniasis .
科学研究应用
Aurachin D has a wide range of scientific research applications:
作用机制
Mode of Action
Aurachin D interacts with its targets, the cytochrome bd oxidases, and inhibits their function . It acts in the vicinity of heme b-558 and prevents the oxidation of substrate quinols and the resulting electron transfer to heme b-558 .
Biochemical Pathways
The inhibition of cytochrome bd oxidases by Aurachin D affects the electron transport chain, a crucial biochemical pathway in bacteria . This disruption can lead to a decrease in ATP production, affecting the energy metabolism of the bacteria and potentially leading to cell death .
Pharmacokinetics
It is known that the compound is produced biocatalytically from a quinolone precursor molecule with recombinant escherichia coli cells expressing the biosynthesis enzyme auaa . The expression of the auaA gene and the introduction of the mevalonate pathway into the production strain can improve the availability of farnesyl pyrophosphate, which is needed as a cosubstrate for the AuaA-catalyzed reaction .
Result of Action
The result of Aurachin D’s action is the inhibition of the bacterial respiratory chain, which can lead to cell death . It has shown remarkable activities against Leishmania donovani, the causative agent of leishmaniasis , and has potential as a treatment for infectious diseases caused by mycobacteria .
Action Environment
The action of Aurachin D can be influenced by environmental factors. For example, the expression of the auaA gene, which is involved in the production of Aurachin D, can be improved using a bicistronic design architecture . Additionally, the introduction of the mevalonate pathway into E. coli stimulates Aurachin D production . These factors can influence the compound’s action, efficacy, and stability.
未来方向
Aurachin D has shown remarkable activities against Leishmania donovani, the causative agent of leishmaniasis . It has received increasing attention as a target for the treatment of infectious diseases caused by mycobacteria . The biotechnological production of Aurachin D was established in E. coli with a titer which is higher than previously reported from natural producer organisms . This suggests that Aurachin D and its derivatives may serve as a new antibiotic class in the future .
生化分析
Biochemical Properties
Aurachin D plays a significant role in biochemical reactions, particularly as an inhibitor of cytochrome bd oxidases . It interacts with these enzymes, inhibiting their function and thereby affecting the electron transport chains within cells .
Cellular Effects
Aurachin D has a profound impact on various types of cells and cellular processes. It influences cell function by inhibiting cytochrome bd oxidases, which are crucial for the survival of pathogenic bacteria during infection and proliferation . This inhibition can affect cell signaling pathways, gene expression, and cellular metabolism .
Molecular Mechanism
The molecular mechanism of Aurachin D involves its interaction with cytochrome bd oxidases . As a selective inhibitor, Aurachin D binds to these enzymes, inhibiting their function and leading to changes in gene expression .
Temporal Effects in Laboratory Settings
The effects of Aurachin D change over time in laboratory settings . The compound’s stability, degradation, and long-term effects on cellular function have been observed in both in vitro and in vivo studies .
Dosage Effects in Animal Models
The effects of Aurachin D vary with different dosages in animal models
Metabolic Pathways
Aurachin D is involved in the metabolic pathways related to the respiratory chain in pro- and eukaryotes . It interacts with enzymes and cofactors within these pathways, potentially affecting metabolic flux or metabolite levels .
准备方法
Synthetic Routes and Reaction Conditions: Aurachin D can be synthesized through several chemical routes. The installation of the farnesyl substituent typically occurs before the formation of the quinolone heterocycle . One common method involves the use of ethyl acetoacetate and substituted aniline derivatives as starting materials . The reaction conditions often include the use of zinc perchlorate hexahydrate and magnesium sulfate in ethanol at room temperature for 12-48 hours .
Industrial Production Methods: Biocatalytic production of Aurachin D has been achieved using recombinant Escherichia coli cells expressing the biosynthesis enzyme AuaA . This approach has significantly increased the titer of Aurachin D compared to traditional chemical synthesis .
化学反应分析
Types of Reactions: Aurachin D undergoes various chemical reactions, including oxidation, reduction, and substitution .
Common Reagents and Conditions:
Oxidation: Common oxidizing agents include potassium permanganate and chromium trioxide.
Reduction: Reducing agents such as sodium borohydride and lithium aluminum hydride are used.
Substitution: Nucleophilic substitution reactions often involve reagents like sodium methoxide and potassium tert-butoxide.
Major Products: The major products formed from these reactions include various derivatives of Aurachin D with modified functional groups, which can exhibit different biological activities .
相似化合物的比较
Similar compounds include Aurachin A, Aurachin B, and Aurachin C . While all these compounds share a common quinolone scaffold, Aurachin D is unique due to its selective inhibition of cytochrome bd oxidase . This specificity makes Aurachin D particularly valuable for studying electron transport processes and developing targeted antibacterial therapies .
属性
IUPAC Name |
2-methyl-3-[(2E,6E)-3,7,11-trimethyldodeca-2,6,10-trienyl]-1H-quinolin-4-one | |
---|---|---|
Source | PubChem | |
URL | https://pubchem.ncbi.nlm.nih.gov | |
Description | Data deposited in or computed by PubChem | |
InChI |
InChI=1S/C25H33NO/c1-18(2)10-8-11-19(3)12-9-13-20(4)16-17-22-21(5)26-24-15-7-6-14-23(24)25(22)27/h6-7,10,12,14-16H,8-9,11,13,17H2,1-5H3,(H,26,27)/b19-12+,20-16+ | |
Source | PubChem | |
URL | https://pubchem.ncbi.nlm.nih.gov | |
Description | Data deposited in or computed by PubChem | |
InChI Key |
JHMLNOXMSHURLQ-YEFHWUCQSA-N | |
Source | PubChem | |
URL | https://pubchem.ncbi.nlm.nih.gov | |
Description | Data deposited in or computed by PubChem | |
Canonical SMILES |
CC1=C(C(=O)C2=CC=CC=C2N1)CC=C(C)CCC=C(C)CCC=C(C)C | |
Source | PubChem | |
URL | https://pubchem.ncbi.nlm.nih.gov | |
Description | Data deposited in or computed by PubChem | |
Isomeric SMILES |
CC1=C(C(=O)C2=CC=CC=C2N1)C/C=C(\C)/CC/C=C(\C)/CCC=C(C)C | |
Source | PubChem | |
URL | https://pubchem.ncbi.nlm.nih.gov | |
Description | Data deposited in or computed by PubChem | |
Molecular Formula |
C25H33NO | |
Source | PubChem | |
URL | https://pubchem.ncbi.nlm.nih.gov | |
Description | Data deposited in or computed by PubChem | |
DSSTOX Substance ID |
DTXSID701318443 | |
Record name | Aurachin D | |
Source | EPA DSSTox | |
URL | https://comptox.epa.gov/dashboard/DTXSID701318443 | |
Description | DSSTox provides a high quality public chemistry resource for supporting improved predictive toxicology. | |
Molecular Weight |
363.5 g/mol | |
Source | PubChem | |
URL | https://pubchem.ncbi.nlm.nih.gov | |
Description | Data deposited in or computed by PubChem | |
CAS No. |
108354-13-8 | |
Record name | Aurachin D | |
Source | CAS Common Chemistry | |
URL | https://commonchemistry.cas.org/detail?cas_rn=108354-13-8 | |
Description | CAS Common Chemistry is an open community resource for accessing chemical information. Nearly 500,000 chemical substances from CAS REGISTRY cover areas of community interest, including common and frequently regulated chemicals, and those relevant to high school and undergraduate chemistry classes. This chemical information, curated by our expert scientists, is provided in alignment with our mission as a division of the American Chemical Society. | |
Explanation | The data from CAS Common Chemistry is provided under a CC-BY-NC 4.0 license, unless otherwise stated. | |
Record name | Aurachin D | |
Source | ChemIDplus | |
URL | https://pubchem.ncbi.nlm.nih.gov/substance/?source=chemidplus&sourceid=0108354138 | |
Description | ChemIDplus is a free, web search system that provides access to the structure and nomenclature authority files used for the identification of chemical substances cited in National Library of Medicine (NLM) databases, including the TOXNET system. | |
Record name | Aurachin D | |
Source | EPA DSSTox | |
URL | https://comptox.epa.gov/dashboard/DTXSID701318443 | |
Description | DSSTox provides a high quality public chemistry resource for supporting improved predictive toxicology. | |
Retrosynthesis Analysis
AI-Powered Synthesis Planning: Our tool employs the Template_relevance Pistachio, Template_relevance Bkms_metabolic, Template_relevance Pistachio_ringbreaker, Template_relevance Reaxys, Template_relevance Reaxys_biocatalysis model, leveraging a vast database of chemical reactions to predict feasible synthetic routes.
One-Step Synthesis Focus: Specifically designed for one-step synthesis, it provides concise and direct routes for your target compounds, streamlining the synthesis process.
Accurate Predictions: Utilizing the extensive PISTACHIO, BKMS_METABOLIC, PISTACHIO_RINGBREAKER, REAXYS, REAXYS_BIOCATALYSIS database, our tool offers high-accuracy predictions, reflecting the latest in chemical research and data.
Strategy Settings
Precursor scoring | Relevance Heuristic |
---|---|
Min. plausibility | 0.01 |
Model | Template_relevance |
Template Set | Pistachio/Bkms_metabolic/Pistachio_ringbreaker/Reaxys/Reaxys_biocatalysis |
Top-N result to add to graph | 6 |
Feasible Synthetic Routes
体外研究产品的免责声明和信息
请注意,BenchChem 上展示的所有文章和产品信息仅供信息参考。 BenchChem 上可购买的产品专为体外研究设计,这些研究在生物体外进行。体外研究,源自拉丁语 "in glass",涉及在受控实验室环境中使用细胞或组织进行的实验。重要的是要注意,这些产品没有被归类为药物或药品,他们没有得到 FDA 的批准,用于预防、治疗或治愈任何医疗状况、疾病或疾病。我们必须强调,将这些产品以任何形式引入人类或动物的身体都是法律严格禁止的。遵守这些指南对确保研究和实验的法律和道德标准的符合性至关重要。