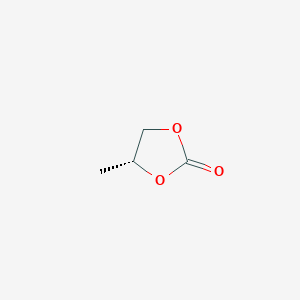
(R)-(+)-Propylene carbonate
概述
描述
®-(+)-Propylene carbonate is a chiral cyclic carbonate with the molecular formula C4H6O3. It is a colorless, odorless, and highly polar solvent known for its high boiling point and low toxicity. This compound is widely used in various industrial applications, including as a solvent in lithium-ion batteries, a plasticizer, and a component in cosmetics and pharmaceuticals.
准备方法
Synthetic Routes and Reaction Conditions: ®-(+)-Propylene carbonate can be synthesized through several methods. One common route involves the reaction of propylene oxide with carbon dioxide in the presence of a catalyst. This reaction typically occurs under mild conditions, such as temperatures ranging from 25°C to 100°C and pressures from 1 to 10 atmospheres. Catalysts used in this process include zinc glutarate, zinc oxide, and various organometallic compounds.
Industrial Production Methods: In industrial settings, ®-(+)-propylene carbonate is produced using continuous flow reactors to ensure high efficiency and yield. The process involves the use of high-pressure reactors and advanced catalysts to facilitate the reaction between propylene oxide and carbon dioxide. The product is then purified through distillation and other separation techniques to achieve the desired purity levels.
化学反应分析
Types of Reactions: ®-(+)-Propylene carbonate undergoes various chemical reactions, including:
Hydrolysis: In the presence of water, ®-(+)-propylene carbonate can hydrolyze to form propylene glycol and carbon dioxide.
Transesterification: This reaction involves the exchange of ester groups between ®-(+)-propylene carbonate and alcohols, leading to the formation of new esters and carbonates.
Polymerization: ®-(+)-Propylene carbonate can polymerize to form polycarbonates, which are used in the production of plastics and resins.
Common Reagents and Conditions:
Hydrolysis: Water and acidic or basic catalysts (e.g., sulfuric acid or sodium hydroxide) are commonly used.
Transesterification: Alcohols (e.g., methanol, ethanol) and catalysts such as sodium methoxide or potassium hydroxide are employed.
Polymerization: Catalysts like zinc chloride or tin compounds are used under controlled temperature and pressure conditions.
Major Products Formed:
Hydrolysis: Propylene glycol and carbon dioxide.
Transesterification: New esters and carbonates.
Polymerization: Polycarbonates.
科学研究应用
®-(+)-Propylene carbonate has a wide range of applications in scientific research, including:
Chemistry: Used as a solvent in various chemical reactions and processes due to its high polarity and stability.
Biology: Employed in the formulation of biological buffers and as a solvent for biological assays.
Medicine: Utilized in pharmaceutical formulations as a solvent and excipient.
Industry: Widely used in the production of lithium-ion batteries, as a plasticizer in polymers, and as a component in cosmetics and personal care products.
作用机制
The mechanism of action of ®-(+)-propylene carbonate primarily involves its role as a solvent. Its high polarity allows it to dissolve a wide range of compounds, facilitating chemical reactions and processes. In lithium-ion batteries, ®-(+)-propylene carbonate acts as an electrolyte solvent, enhancing the conductivity and stability of the electrolyte solution. Its molecular structure allows it to interact with various molecular targets and pathways, promoting efficient ion transport and chemical stability.
相似化合物的比较
Ethylene carbonate: Another cyclic carbonate with similar solvent properties but a different molecular structure.
Dimethyl carbonate: A linear carbonate with lower polarity and different reactivity.
Propylene glycol: A diol with similar applications but different chemical properties.
Uniqueness: ®-(+)-Propylene carbonate stands out due to its chiral nature, high polarity, and low toxicity. Its ability to act as a solvent in a wide range of applications, from batteries to pharmaceuticals, makes it a versatile and valuable compound in various industries.
属性
IUPAC Name |
(4R)-4-methyl-1,3-dioxolan-2-one | |
---|---|---|
Source | PubChem | |
URL | https://pubchem.ncbi.nlm.nih.gov | |
Description | Data deposited in or computed by PubChem | |
InChI |
InChI=1S/C4H6O3/c1-3-2-6-4(5)7-3/h3H,2H2,1H3/t3-/m1/s1 | |
Source | PubChem | |
URL | https://pubchem.ncbi.nlm.nih.gov | |
Description | Data deposited in or computed by PubChem | |
InChI Key |
RUOJZAUFBMNUDX-GSVOUGTGSA-N | |
Source | PubChem | |
URL | https://pubchem.ncbi.nlm.nih.gov | |
Description | Data deposited in or computed by PubChem | |
Canonical SMILES |
CC1COC(=O)O1 | |
Source | PubChem | |
URL | https://pubchem.ncbi.nlm.nih.gov | |
Description | Data deposited in or computed by PubChem | |
Isomeric SMILES |
C[C@@H]1COC(=O)O1 | |
Source | PubChem | |
URL | https://pubchem.ncbi.nlm.nih.gov | |
Description | Data deposited in or computed by PubChem | |
Molecular Formula |
C4H6O3 | |
Source | PubChem | |
URL | https://pubchem.ncbi.nlm.nih.gov | |
Description | Data deposited in or computed by PubChem | |
DSSTOX Substance ID |
DTXSID901301204 | |
Record name | (4R)-4-Methyl-1,3-dioxolan-2-one | |
Source | EPA DSSTox | |
URL | https://comptox.epa.gov/dashboard/DTXSID901301204 | |
Description | DSSTox provides a high quality public chemistry resource for supporting improved predictive toxicology. | |
Molecular Weight |
102.09 g/mol | |
Source | PubChem | |
URL | https://pubchem.ncbi.nlm.nih.gov | |
Description | Data deposited in or computed by PubChem | |
CAS No. |
16606-55-6 | |
Record name | (4R)-4-Methyl-1,3-dioxolan-2-one | |
Source | CAS Common Chemistry | |
URL | https://commonchemistry.cas.org/detail?cas_rn=16606-55-6 | |
Description | CAS Common Chemistry is an open community resource for accessing chemical information. Nearly 500,000 chemical substances from CAS REGISTRY cover areas of community interest, including common and frequently regulated chemicals, and those relevant to high school and undergraduate chemistry classes. This chemical information, curated by our expert scientists, is provided in alignment with our mission as a division of the American Chemical Society. | |
Explanation | The data from CAS Common Chemistry is provided under a CC-BY-NC 4.0 license, unless otherwise stated. | |
Record name | (4R)-4-Methyl-1,3-dioxolan-2-one | |
Source | EPA DSSTox | |
URL | https://comptox.epa.gov/dashboard/DTXSID901301204 | |
Description | DSSTox provides a high quality public chemistry resource for supporting improved predictive toxicology. | |
Record name | 1,3-Dioxolan-2-one, 4-methyl-, (4R) | |
Source | European Chemicals Agency (ECHA) | |
URL | https://echa.europa.eu/substance-information/-/substanceinfo/100.120.807 | |
Description | The European Chemicals Agency (ECHA) is an agency of the European Union which is the driving force among regulatory authorities in implementing the EU's groundbreaking chemicals legislation for the benefit of human health and the environment as well as for innovation and competitiveness. | |
Explanation | Use of the information, documents and data from the ECHA website is subject to the terms and conditions of this Legal Notice, and subject to other binding limitations provided for under applicable law, the information, documents and data made available on the ECHA website may be reproduced, distributed and/or used, totally or in part, for non-commercial purposes provided that ECHA is acknowledged as the source: "Source: European Chemicals Agency, http://echa.europa.eu/". Such acknowledgement must be included in each copy of the material. ECHA permits and encourages organisations and individuals to create links to the ECHA website under the following cumulative conditions: Links can only be made to webpages that provide a link to the Legal Notice page. | |
Synthesis routes and methods I
Procedure details
Synthesis routes and methods II
Procedure details
Synthesis routes and methods III
Procedure details
Retrosynthesis Analysis
AI-Powered Synthesis Planning: Our tool employs the Template_relevance Pistachio, Template_relevance Bkms_metabolic, Template_relevance Pistachio_ringbreaker, Template_relevance Reaxys, Template_relevance Reaxys_biocatalysis model, leveraging a vast database of chemical reactions to predict feasible synthetic routes.
One-Step Synthesis Focus: Specifically designed for one-step synthesis, it provides concise and direct routes for your target compounds, streamlining the synthesis process.
Accurate Predictions: Utilizing the extensive PISTACHIO, BKMS_METABOLIC, PISTACHIO_RINGBREAKER, REAXYS, REAXYS_BIOCATALYSIS database, our tool offers high-accuracy predictions, reflecting the latest in chemical research and data.
Strategy Settings
Precursor scoring | Relevance Heuristic |
---|---|
Min. plausibility | 0.01 |
Model | Template_relevance |
Template Set | Pistachio/Bkms_metabolic/Pistachio_ringbreaker/Reaxys/Reaxys_biocatalysis |
Top-N result to add to graph | 6 |
Feasible Synthetic Routes
体外研究产品的免责声明和信息
请注意,BenchChem 上展示的所有文章和产品信息仅供信息参考。 BenchChem 上可购买的产品专为体外研究设计,这些研究在生物体外进行。体外研究,源自拉丁语 "in glass",涉及在受控实验室环境中使用细胞或组织进行的实验。重要的是要注意,这些产品没有被归类为药物或药品,他们没有得到 FDA 的批准,用于预防、治疗或治愈任何医疗状况、疾病或疾病。我们必须强调,将这些产品以任何形式引入人类或动物的身体都是法律严格禁止的。遵守这些指南对确保研究和实验的法律和道德标准的符合性至关重要。