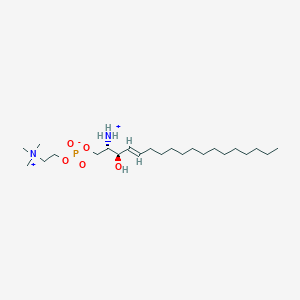
Sphingosylphosphorylcholine
描述
Sphingosylphosphorylcholine is a naturally occurring bioactive sphingolipid found in blood plasma. It is metabolized from the hydrolysis of membrane sphingolipids and is composed of a long-chain sphingosine and phosphorylcholine . This compound shares structural and functional similarities with other sphingolipids such as ceramide, sphingosine-1-phosphate, and lysosphingolipids . It plays a crucial role in various cellular processes, including cell differentiation, proliferation, and apoptosis .
准备方法
Synthetic Routes and Reaction Conditions
Sphingosylphosphorylcholine can be synthesized through the hydrolysis of sphingomyelin by sphingomyelinase or sphingomyelin deacylase . The reaction conditions typically involve the use of specific enzymes that catalyze the hydrolysis process. The production of this compound from sphingomyelin is a precisely regulated enzymatic process .
Industrial Production Methods
Industrial production of this compound involves the extraction and purification of the compound from biological sources such as blood plasma or platelets . The process includes the use of chromatographic techniques to isolate and purify this compound from other sphingolipids and lipoproteins .
化学反应分析
Types of Reactions
Sphingosylphosphorylcholine undergoes various chemical reactions, including:
Oxidation: this compound can be oxidized to form different metabolites.
Reduction: Reduction reactions can modify the functional groups of this compound.
Substitution: This compound can participate in substitution reactions where functional groups are replaced by other groups.
Common Reagents and Conditions
Common reagents used in the reactions of this compound include oxidizing agents, reducing agents, and catalysts that facilitate substitution reactions . The reaction conditions vary depending on the desired product and the specific reaction being carried out.
Major Products Formed
The major products formed from the reactions of this compound include various metabolites that play significant roles in cellular processes . These products can influence cell signaling pathways and physiological functions.
科学研究应用
Sphingosylphosphorylcholine has a wide range of scientific research applications, including:
Chemistry: this compound is studied for its chemical properties and reactions.
Biology: It plays a role in cell differentiation, proliferation, and apoptosis.
Medicine: This compound is involved in the modulation of cardiovascular functions and diseases.
Industry: This compound is used in the development of pharmaceuticals and therapeutic agents.
作用机制
Sphingosylphosphorylcholine exerts its effects through various molecular targets and pathways. It can act as a first messenger through G protein-coupled receptors or membrane lipid rafts, or as a second messenger mediating intracellular calcium release . The compound influences the functions of myocytes, vein endothelial cells, and vascular smooth muscle cells, thereby modulating cardiovascular functions . Additionally, this compound can activate pathways such as the Parkin and nuclear receptor subfamily group A member 2/optineurin pathways, leading to increased levels of mitophagy in affected myocardium .
相似化合物的比较
Sphingosylphosphorylcholine is similar to other sphingolipids such as ceramide, sphingosine-1-phosphate, and lysosphingolipids . it is unique in its ability to act as both a first and second messenger, influencing a wide range of cellular processes . Other similar compounds include:
Ceramide: Involved in cell signaling and apoptosis.
Sphingosine-1-phosphate: Plays a role in cell proliferation and migration.
Lysosphingolipids: Involved in various cellular functions and signaling pathways.
This compound’s unique properties and functions make it a valuable compound in scientific research and therapeutic applications.
生物活性
Sphingosylphosphorylcholine (SPC) is a unique lysosphingolipid that exhibits a wide range of biological activities, influencing various physiological and pathological processes. This article delves into the mechanisms of action, effects on different cell types, and its implications in diseases, particularly cancer and inflammation.
SPC is derived from sphingomyelin through the action of sphingomyelin deacylase. It is present in blood and has been associated with several diseases, including cancer, atopic dermatitis, and Niemann-Pick disease (NPD) . Elevated levels of SPC have been observed in various pathological conditions, indicating its role as a potential biomarker and therapeutic target.
SPC exerts its biological effects primarily through interaction with specific receptors and modulation of intracellular signaling pathways:
- Receptor Activation : SPC can activate sphingosine-1-phosphate (S1P) receptors (S1P1-S1P5), leading to various cellular responses such as proliferation, migration, and differentiation .
- Calcium Mobilization : SPC has been shown to induce calcium release from the endoplasmic reticulum, resulting in acute elevations in cytosolic calcium levels. This effect is critical for various cellular functions, including muscle contraction and neurotransmitter release .
3. Biological Activities
The biological activities of SPC can be categorized based on its effects on different cell types:
3.1 Cancer Cells
SPC has been extensively studied for its role in cancer biology:
- Inhibition of Cell Growth : In neuroblastoma cell lines (NS-20Y, Neuro2a, N1E-115), SPC inhibited growth at concentrations below 150 µM while promoting neurite outgrowth .
- Impact on Tumor Microenvironment : SPC influences the tumor microenvironment by modulating immune cell functions and promoting angiogenesis .
Cell Type | Concentration | Effect | Mechanism |
---|---|---|---|
Mouse Neuroblastoma Cells | < 150 µM | Growth inhibition; neurite outgrowth | Sphingomyelin metabolism |
Human Bladder Carcinoma Cells | 10 µM | Migration and invasion decrease | GPCR signaling |
Anaplastic Thyroid Carcinoma | 1-10 µM | Proliferation and migration decrease | PI3K-Akt pathway |
3.2 Immune System
SPC plays a significant role in modulating immune responses:
- Inhibition of Plasma Cell Differentiation : Recent studies indicate that SPC inhibits the differentiation of B cells into plasma cells, potentially influencing autoimmune conditions such as multiple sclerosis .
- Regulation of Inflammatory Responses : Elevated levels of SPC are linked to increased inflammatory responses in conditions like atopic dermatitis .
4. Case Studies
Several case studies highlight the relevance of SPC in clinical settings:
- Atopic Dermatitis : A study found that SPC levels were significantly elevated in the stratum corneum of patients with atopic dermatitis compared to healthy controls. This increase correlated with decreased ceramide levels, suggesting a role for SPC in skin barrier dysfunction .
- Cancer Progression : In ovarian cancer patients, higher levels of SPC were detected in ascitic fluid, indicating its potential as a marker for disease progression .
5. Conclusion
This compound is a multifaceted lipid mediator with significant implications for various biological processes. Its ability to modulate cell proliferation, migration, and differentiation makes it a crucial player in both normal physiology and pathological conditions. Ongoing research into its mechanisms may pave the way for novel therapeutic strategies targeting SPC-related pathways.
属性
IUPAC Name |
(2-amino-3-hydroxyoctadec-4-enyl) 2-(trimethylazaniumyl)ethyl phosphate | |
---|---|---|
Details | Computed by LexiChem 2.6.6 (PubChem release 2019.06.18) | |
Source | PubChem | |
URL | https://pubchem.ncbi.nlm.nih.gov | |
Description | Data deposited in or computed by PubChem | |
InChI |
InChI=1S/C23H49N2O5P/c1-5-6-7-8-9-10-11-12-13-14-15-16-17-18-23(26)22(24)21-30-31(27,28)29-20-19-25(2,3)4/h17-18,22-23,26H,5-16,19-21,24H2,1-4H3 | |
Details | Computed by InChI 1.0.5 (PubChem release 2019.06.18) | |
Source | PubChem | |
URL | https://pubchem.ncbi.nlm.nih.gov | |
Description | Data deposited in or computed by PubChem | |
InChI Key |
JLVSPVFPBBFMBE-UHFFFAOYSA-N | |
Details | Computed by InChI 1.0.5 (PubChem release 2019.06.18) | |
Source | PubChem | |
URL | https://pubchem.ncbi.nlm.nih.gov | |
Description | Data deposited in or computed by PubChem | |
Canonical SMILES |
CCCCCCCCCCCCCC=CC(C(COP(=O)([O-])OCC[N+](C)(C)C)N)O | |
Details | Computed by OEChem 2.1.5 (PubChem release 2019.06.18) | |
Source | PubChem | |
URL | https://pubchem.ncbi.nlm.nih.gov | |
Description | Data deposited in or computed by PubChem | |
Molecular Formula |
C23H49N2O5P | |
Details | Computed by PubChem 2.1 (PubChem release 2019.06.18) | |
Source | PubChem | |
URL | https://pubchem.ncbi.nlm.nih.gov | |
Description | Data deposited in or computed by PubChem | |
DSSTOX Substance ID |
DTXSID40274460 | |
Record name | 2-Amino-3-hydroxyoctadec-4-en-1-yl 2-(trimethylazaniumyl)ethyl phosphate | |
Source | EPA DSSTox | |
URL | https://comptox.epa.gov/dashboard/DTXSID40274460 | |
Description | DSSTox provides a high quality public chemistry resource for supporting improved predictive toxicology. | |
Molecular Weight |
464.6 g/mol | |
Details | Computed by PubChem 2.1 (PubChem release 2021.05.07) | |
Source | PubChem | |
URL | https://pubchem.ncbi.nlm.nih.gov | |
Description | Data deposited in or computed by PubChem | |
CAS No. |
1670-26-4 | |
Record name | 2-Amino-3-hydroxyoctadec-4-en-1-yl 2-(trimethylazaniumyl)ethyl phosphate | |
Source | EPA DSSTox | |
URL | https://comptox.epa.gov/dashboard/DTXSID40274460 | |
Description | DSSTox provides a high quality public chemistry resource for supporting improved predictive toxicology. | |
Retrosynthesis Analysis
AI-Powered Synthesis Planning: Our tool employs the Template_relevance Pistachio, Template_relevance Bkms_metabolic, Template_relevance Pistachio_ringbreaker, Template_relevance Reaxys, Template_relevance Reaxys_biocatalysis model, leveraging a vast database of chemical reactions to predict feasible synthetic routes.
One-Step Synthesis Focus: Specifically designed for one-step synthesis, it provides concise and direct routes for your target compounds, streamlining the synthesis process.
Accurate Predictions: Utilizing the extensive PISTACHIO, BKMS_METABOLIC, PISTACHIO_RINGBREAKER, REAXYS, REAXYS_BIOCATALYSIS database, our tool offers high-accuracy predictions, reflecting the latest in chemical research and data.
Strategy Settings
Precursor scoring | Relevance Heuristic |
---|---|
Min. plausibility | 0.01 |
Model | Template_relevance |
Template Set | Pistachio/Bkms_metabolic/Pistachio_ringbreaker/Reaxys/Reaxys_biocatalysis |
Top-N result to add to graph | 6 |
Feasible Synthetic Routes
Q1: How does SPC interact with its targets?
A1: SPC interacts with various targets, including G protein-coupled receptors (GPCRs) and intracellular proteins like calmodulin.
- GPCR Interaction: SPC binds to specific GPCRs, such as GPR12 [], EDG1, EDG3, and AGR16 [], triggering downstream signaling cascades. This interaction is often pertussis toxin-sensitive, indicating the involvement of Gi/o proteins [, , ].
- Calmodulin Interaction: SPC directly binds to both apo- and Ca2+-saturated calmodulin [, ], inhibiting its interaction with target proteins like calcineurin and phosphodiesterase, thereby modulating calcium signaling pathways [].
Q2: What are the downstream effects of SPC binding to its targets?
A2: SPC elicits diverse cellular responses depending on the cell type and the specific target engaged. These effects include:
- Cell Proliferation and Differentiation: SPC stimulates the proliferation of various cell types, including human adipose tissue-derived mesenchymal stem cells (hADSCs) [], and induces their differentiation into smooth muscle cells (SMCs) through a TGF-β-dependent mechanism [, ].
- Cell Migration and Invasion: SPC enhances the migration and invasion of cancer cells, potentially contributing to metastasis. This is partly mediated by keratin 8 (K8) phosphorylation and reorganization, leading to increased cell viscoelasticity [, , ], and by the secretion of matrix metalloproteinase-3 (MMP-3) [].
- Apoptosis: SPC can induce apoptosis in specific cell types, such as Neuro 2a cells [] and breast cancer cells [], through the activation of different protein kinase C (PKC) isozymes and mitochondrial pathways [].
- Calcium Signaling: SPC triggers a rapid and transient increase in intracellular calcium concentration ([Ca2+]i) in various cells, including porcine aortic smooth muscle cells [, ], human airway epithelial cells [], and rat glomerular mesangial cells []. This increase can be mediated by both Ca2+ release from intracellular stores and Ca2+ influx from the extracellular space [].
Q3: What is the molecular formula and weight of SPC?
A3: The molecular formula of SPC is C26H55N2O8P, and its molecular weight is 554.7 g/mol.
Q4: Is there spectroscopic data available for SPC?
A4: Yes, spectroscopic data like 1H and 13C NMR, Fourier transform infrared spectrometry, and mass spectrometry have been used to identify and characterize SPC and related lipids [].
Q5: What is known about the stability of SPC?
A5: While detailed stability studies under various conditions were not presented, the research suggests that:
- SPC levels can be measured in biological samples: Researchers quantified SPC in biological fluids like cerebrospinal fluid (CSF) [], suggesting its stability during sample processing and analysis.
Q6: How can computational chemistry be applied to further understand SPC?
A6: Computational chemistry can be instrumental in:
Q7: How do structural modifications of SPC affect its activity?
A7: Limited SAR information is available from the provided research, but some observations include:
- Stereochemistry: The D-erythro stereoisomer of SPC was found to be more potent than the L-threo isomer in stimulating transmitter release from frog motor nerve endings [].
- Acylation: N-acylated SPC derivatives showed distinct chromatographic behavior compared to the parent compound [], suggesting a potential impact of acylation on its physicochemical properties and potentially its activity.
- Chain Length and Saturation: The enzyme NPP6, which hydrolyzes SPC, displayed a preference for lysophosphatidylcholine (LPC) substrates with short or polyunsaturated fatty acid chains []. This suggests that the fatty acid moiety of SPC may influence its interaction with certain enzymes and potentially its biological activity.
Q8: What is known about the pharmacokinetics of SPC?
A8: While detailed ADME studies were not presented, the research indicates that:
- SPC can be administered intravenously: Intravenous administration of SPC was shown to be effective in reducing infarct size in a mouse model of myocardial ischemia/reperfusion injury [], suggesting its absorption and distribution to target tissues.
- SPC is present in various biological fluids: The detection of SPC in plasma [] and CSF [] indicates its distribution to these compartments.
Q9: What are the pharmacodynamic effects of SPC?
A9: The pharmacodynamic effects of SPC are diverse and depend on the target cell and the specific signaling pathways activated. Key pharmacodynamic observations include:
- SPC induces vasoconstriction in cerebral arteries: This effect involves the activation of Rho kinase and Src family protein tyrosine kinases (Src-PTKs), leading to Ca2+ sensitization of vascular smooth muscle contraction [, ].
- SPC protects against myocardial reperfusion injury: This cardioprotective effect is mediated by the S1P3 receptor and involves reduced neutrophil recruitment, attenuated apoptosis, and inhibition of leukocyte adhesion to endothelial cells [].
- SPC modulates bone metabolism: SPC inhibits osteoclast differentiation and bone resorption, potentially by suppressing Ca2+/calmodulin-mediated signaling pathways [].
Q10: What in vitro models have been used to study SPC?
A10: Researchers have employed various in vitro models, including:
- Cultured cell lines: Studies utilized cell lines like Swiss 3T3 fibroblasts [, ], porcine aortic smooth muscle cells [, ], human airway epithelial cells [], rat glomerular mesangial cells [], Neuro 2a cells [], breast cancer cell lines (e.g., MDA-MB-231) [], pancreatic cancer cell lines (e.g., PANC-1) [, , , ], and human melanocytes [, ].
- Primary cell cultures: Studies used primary cultures of rat aortic vascular smooth muscle cells [] and human macrophages [].
- Cell-populated collagen gel fibers: This model was used to study the effects of SPC on the contraction of vascular smooth muscle cells [].
Q11: What in vivo models have been used to study SPC?
A11: Researchers utilized animal models including:
- Mouse model of myocardial ischemia/reperfusion injury: This model was used to evaluate the cardioprotective effects of SPC [].
- Canine model of cerebral vasospasm: Researchers investigated the effects of SPC on cerebral vasospasm induced by subarachnoid hemorrhage (SAH) [].
- Experimental autoimmune encephalomyelitis (EAE) mouse model: This model was used to study the effects of SPC on MS-like disease progression [].
- Ovariectomized mouse model of osteoporosis: This model was used to evaluate the effects of SPC on bone loss [].
- Rat model of endotoxemia: Researchers investigated the anti-inflammatory effects of SPC in endotoxin-induced organ injury [].
Q12: Are there any known mechanisms of resistance to SPC?
A12: While specific resistance mechanisms have not been fully elucidated, potential mechanisms could involve:
- Increased expression of SPC-degrading enzymes: Elevated levels of enzymes like NPP6, which hydrolyzes SPC [], could potentially reduce its effective concentration and diminish its effects.
Q13: What strategies could be employed to improve SPC delivery to specific targets?
A13: Targeted drug delivery strategies for SPC could involve:
Q14: What are potential biomarkers for monitoring SPC's effects?
A14: Potential biomarkers for monitoring SPC's effects could include:
Q15: What analytical methods are used to characterize and quantify SPC?
A15: Several analytical techniques have been employed for SPC analysis, including:
- High-performance liquid chromatography (HPLC): HPLC, often coupled with mass spectrometry (MS), provides sensitive and specific quantification of SPC in biological samples [].
- Mass Spectrometry (MS): MS techniques, such as triple quadrupole MS [], enable accurate and sensitive detection and quantification of SPC and its metabolites.
- Thin Layer Chromatography (TLC): TLC is a simpler method for separating and analyzing lipids, including SPC and its derivatives [].
Q16: How is SPC extracted from biological samples for analysis?
A16: Solid-phase extraction (SPE) is a common technique for extracting and purifying SPC from biological matrices like CSF before analysis [].
Q17: Are there any known alternatives or substitutes for SPC?
A17: Several compounds share some functional similarities with SPC, including:
Q18: How do these alternatives compare to SPC in terms of activity?
A18: The specific activities of SPC, S1P, and LPA are distinct and depend on the cellular context and the receptors and downstream signaling pathways involved.
- Cerebral Vasospasm: SPC and S1P can both induce vasoconstriction in cerebral arteries through Rho kinase activation [].
- Cardioprotection: While both SPC and S1P have been implicated in cardioprotection, they may act through different S1P receptor subtypes [].
- Osteoclast Differentiation: SPC inhibits osteoclast differentiation [], whereas S1P has been shown to promote osteoclastogenesis indirectly by stimulating osteoblasts [].
体外研究产品的免责声明和信息
请注意,BenchChem 上展示的所有文章和产品信息仅供信息参考。 BenchChem 上可购买的产品专为体外研究设计,这些研究在生物体外进行。体外研究,源自拉丁语 "in glass",涉及在受控实验室环境中使用细胞或组织进行的实验。重要的是要注意,这些产品没有被归类为药物或药品,他们没有得到 FDA 的批准,用于预防、治疗或治愈任何医疗状况、疾病或疾病。我们必须强调,将这些产品以任何形式引入人类或动物的身体都是法律严格禁止的。遵守这些指南对确保研究和实验的法律和道德标准的符合性至关重要。