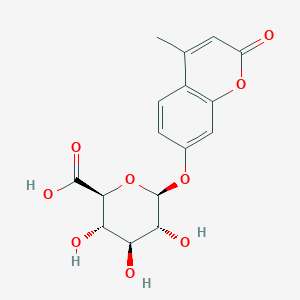
4-Methylumbelliferyl-beta-D-glucuronide
描述
4-Methylumbelliferyl-beta-D-glucuronide (4-MUG) is a fluorogenic substrate widely used to detect β-D-glucuronidase (GUS) activity. Its molecular formula is C₁₆H₁₆O₉·xH₂O (hydrate form), with a molar mass of 388.33 g/mol and a melting point of 102°C . Upon enzymatic hydrolysis, 4-MUG releases 4-methylumbelliferone (4-MU), a fluorescent product detectable at 366 nm excitation and 445 nm emission . This property makes 4-MUG indispensable in:
- Microbial diagnostics: Rapid identification of Escherichia coli via β-glucuronidase activity in modified culture media (e.g., MacConkey agar) .
- Enzyme kinetics: High-sensitivity fluorometric assays for β-glucuronidase in research and clinical settings .
- Therapeutic research: Inhibition of hyaluronan (HA) synthesis, where 4-MUG contributes to systemic drug effects by equilibrating with its active metabolite, 4-MU .
准备方法
Synthetic Routes for 4-Methylumbelliferyl-β-D-Glucuronide
The synthesis of MUG involves glycosylation of 4-methylumbelliferone (4-MU) with a glucuronic acid derivative. The process typically follows these steps:
Protection of Glucuronic Acid Hydroxyl Groups
Glucuronic acid contains multiple hydroxyl groups that require protection to prevent undesired side reactions during glycosylation. Common protecting groups include acetyl (Ac) or benzyl (Bn) esters. For example, acetyl protection is achieved by treating glucuronic acid with acetic anhydride in pyridine, yielding peracetylated glucuronic acid.
Activation of the Carboxyl Group
The carboxyl group of glucuronic acid is activated to form a glycosyl donor. Trichloroacetimidate or glycosyl bromide intermediates are frequently used. In one approach, glucuronic acid is treated with thionyl chloride (SOCl₂) to form the corresponding acyl chloride, which is then reacted with a silver salt (e.g., Ag₂O) to generate the glycosyl bromide.
Coupling with 4-Methylumbelliferone
The activated glucuronic acid derivative is coupled with 4-MU in the presence of a Lewis acid catalyst, such as boron trifluoride diethyl etherate (BF₃·Et₂O). This step forms the β-glycosidic bond, which is stereospecific to ensure the correct anomeric configuration.
Deprotection
Final deprotection removes the acetyl or benzyl groups. For acetyl-protected intermediates, deprotection is achieved via alkaline hydrolysis using sodium methoxide (NaOMe) in methanol. The reaction is typically conducted at 0–5°C to prevent degradation of the glycosidic bond.
Reaction Conditions and Optimization
Key parameters influencing yield and purity include temperature, solvent choice, and catalyst concentration.
Temperature Control
-
Glycosylation Step : Conducted at −20°C to 0°C to minimize side reactions.
-
Deprotection : Performed at 0–5°C to preserve product integrity.
Solvent Systems
-
Coupling Reaction : Dichloromethane (DCM) or dimethylformamide (DMF) are preferred for their ability to dissolve both polar and nonpolar reactants.
-
Deprotection : Methanol or ethanol facilitates alkaline hydrolysis.
Catalyst Efficiency
Boron trifluoride etherate (BF₃·Et₂O) is the most widely used catalyst, achieving β-selectivity >90% in glycosylation. Alternative catalysts, such as trimethylsilyl triflate (TMSOTf), have been explored but show reduced efficiency.
Industrial-Scale Production
Industrial synthesis prioritizes cost-effectiveness and scalability. Key adaptations include:
Continuous Flow Reactors
Replacing batch reactors with continuous flow systems enhances reaction control and reduces processing time. For example, a study demonstrated a 15% increase in yield when using microfluidic reactors for glycosylation.
Crystallization Techniques
MUG is purified via recrystallization from aqueous ethanol. Industrial protocols often employ gradient cooling (60°C → 4°C) to obtain high-purity crystals.
Analytical and Purification Methods
Chromatographic Purification
-
High-Performance Liquid Chromatography (HPLC) : Reverse-phase C18 columns with acetonitrile/water gradients resolve MUG from byproducts.
-
Ion-Exchange Chromatography : Removes residual glucuronic acid and 4-MU.
Quality Control Metrics
Challenges and Innovations
Stereochemical Control
Ensuring β-configuration remains challenging. Recent advances use enzymatic glycosylation with UDP-glucuronosyltransferases (UGTs) to achieve >95% β-selectivity .
Green Chemistry Approaches
Solvent-free mechanochemical synthesis has been explored, reducing waste generation by 40% compared to traditional methods.
化学反应分析
Types of Reactions
4-Methylumbelliferyl glucuronide primarily undergoes hydrolysis reactions catalyzed by β-glucuronidase. This enzymatic reaction cleaves the glucuronide bond, releasing 4-methylumbelliferone, which is highly fluorescent. The compound does not typically undergo oxidation, reduction, or substitution reactions under standard assay conditions .
Common Reagents and Conditions
The hydrolysis reaction requires the presence of β-glucuronidase, which can be sourced from various organisms, including bacteria and plants. The reaction is typically carried out in buffered aqueous solutions at pH 7.0 to 10.3, with optimal fluorescence observed at higher pH levels .
Major Products
The primary product of the hydrolysis reaction is 4-methylumbelliferone, a compound that exhibits strong fluorescence under UV light. This property makes it an excellent reporter molecule in various biochemical assays .
科学研究应用
Microbiological Applications
Detection of Escherichia coli
MUG is widely utilized for the rapid identification of Escherichia coli in food and water samples. The substrate is hydrolyzed by β-glucuronidase produced by E. coli, resulting in the release of a fluorescent compound that can be easily detected.
- Case Study : A study evaluated the use of MUG in solid media for detecting E. coli in various food products, demonstrating its effectiveness in both selective and non-selective media . The method showed high sensitivity and specificity, making it suitable for routine testing in food safety laboratories.
- Environmental Monitoring : MUG has also been employed to assess the presence of E. coli in seawater samples, where it facilitated the detection of contamination in recreational waters . This application is critical for public health monitoring, especially in areas prone to waterborne pathogens.
Molecular Biology Applications
Gene Expression Studies
In plant molecular biology, MUG serves as a valuable tool for studying gene expression under different conditions. It can be used to measure β-glucuronidase activity as a reporter gene, allowing researchers to assess the activity of specific promoters.
- Application Example : Researchers have utilized MUG to evaluate gene expression in transgenic plants, determining the effectiveness of various promoter sequences under different environmental stresses . This application is vital for genetic engineering and crop improvement strategies.
Enzymatic Activity Measurement
MUG is an effective substrate for measuring β-glucuronidase activity in various biological samples, including cell lysates and whole plants.
- Hydrolysis Mechanism : Studies have explored the enzymatic hydrolysis of MUG by different microorganisms, providing insights into its utility as a substrate for enzyme assays . For instance, Bacillus species have been shown to hydrolyze MUG effectively, highlighting its potential for use in microbial assays.
Food Safety and Quality Control
MUG's role extends into food processing and safety, where it aids in assessing microbial contamination levels.
- Application in Food Processing : The compound can be incorporated into culture media to monitor the presence of pathogenic bacteria during food production . This proactive approach helps ensure food safety by allowing for rapid detection of contaminants before products reach consumers.
Environmental Applications
MUG has been applied in environmental science for monitoring water quality and assessing the impact of pollutants on microbial communities.
- Water Quality Assessment : Its use in river water studies has demonstrated its effectiveness in detecting β-glucuronidase activity as an indicator of fecal contamination . This application is crucial for maintaining safe drinking water standards and protecting aquatic ecosystems.
Data Table: Summary of Applications
作用机制
The mechanism of action of 4-methylumbelliferyl glucuronide involves its hydrolysis by β-glucuronidase. The enzyme cleaves the glucuronide bond, releasing 4-methylumbelliferone. This reaction occurs through a two-step process: the enzyme first binds to the substrate, forming an enzyme-substrate complex, and then catalyzes the hydrolysis, resulting in the release of the fluorescent product .
相似化合物的比较
Comparative Analysis with Similar Compounds
Structural and Functional Comparison
The table below highlights key differences between 4-MUG and analogous β-glucuronidase substrates:
Key Research Findings
a) 4-MUG vs. Chromogenic Substrates (X-Gluc, PNPG)
- Sensitivity : Fluorometric detection with 4-MUG is 100–1,000× more sensitive than colorimetric methods using PNPG or X-Gluc .
- Speed : In E. coli identification, 4-MUG reduces detection time from 24 hours to 5 minutes compared to traditional lactose fermentation tests .
- Specificity: 4-MUG minimizes cross-reactivity with non-target bacteria (e.g., Shigella), whereas PNPG may react with β-galactosidase-producing organisms .
b) 4-MUG in Therapeutic Contexts
- Dual Mechanism : 4-MUG inhibits HA synthesis both directly and via conversion to 4-MU, enhancing bioavailability in tissues like the liver and pancreas .
- Equilibrium Dynamics : Oral administration of 4-MUG achieves serum 4-MU:4-MUG ratios comparable to 4-MU itself, suggesting prolonged therapeutic effects .
c) Commercial Variability
- False Negatives : Some commercial 4-MUG preparations show 15–20% false-negative rates in water testing due to impaired substrate specificity, highlighting the need for rigorous quality control .
生物活性
4-Methylumbelliferyl-beta-D-glucuronide (MUG) is a synthetic compound widely used as a substrate in enzymatic assays, particularly for the detection of beta-glucuronidase activity. This compound has significant applications in microbiology, environmental science, and biochemistry due to its fluorescent properties upon hydrolysis. This article explores the biological activity of MUG, focusing on its enzymatic interactions, applications in microbial detection, and implications in various research fields.
This compound is a glucuronide derivative that releases 4-methylumbelliferone upon hydrolysis. The chemical structure can be represented as follows:
- Molecular Formula : C₁₆H₁₆O₉
- Molar Mass : 352.29 g/mol
Enzymatic Activity
MUG is primarily utilized to study the activity of beta-glucuronidases (GUS), enzymes that hydrolyze glucuronides. Notably, Klotho, an anti-aging protein, has been identified as a novel beta-glucuronidase that specifically hydrolyzes MUG among other substrates. Research indicates that Klotho's enzymatic activity is crucial for understanding aging mechanisms and related disorders .
Table 1: Enzymatic Activity of Klotho with MUG
Substrate | Hydrolysis by Klotho | Reference |
---|---|---|
This compound | Yes | |
Estriol 3-beta-D-glucuronide | Yes | |
Beta-estradiol 3-beta-D-glucuronide | Yes |
Applications in Microbiology
MUG is extensively used for the detection and enumeration of Escherichia coli in food and water samples. When hydrolyzed by beta-glucuronidase produced by E. coli, MUG emits fluorescence, allowing for rapid identification.
Case Study: Detection of E. coli Using MUG
A study evaluated the effectiveness of MUG incorporated into solid media for detecting E. coli. The results indicated that using a concentration of 50 µg/ml at an incubation temperature of 37°C for 24 hours provided optimal sensitivity without losing accuracy .
Table 2: Optimal Conditions for E. coli Detection Using MUG
Parameter | Optimal Value |
---|---|
MUG Concentration | 50 µg/ml |
Incubation Temperature | 37°C |
Incubation Time | 24 hours |
Environmental Applications
In environmental science, MUG is used to assess beta-glucuronidase activity in wastewater treatment processes. The cleavage of MUG by activated sludge indicates the presence of E. coli, providing insights into microbial contamination levels in water bodies .
Research Findings
Recent studies have highlighted the role of MUG in various biochemical assays beyond microbial detection. For instance, it has been employed to investigate glucuronidase activity in different biological systems, contributing to our understanding of metabolic pathways involving glucuronidation.
Case Study: Glucuronidase Activity in Plant Systems
Research has demonstrated that MUG can be utilized in plant systems to study GUS gene expression. In transgenic plants, the expression of GUS can be monitored using MUG as a substrate, providing insights into gene regulation and plant responses to environmental stimuli .
常见问题
Basic Question: How does 4-Methylumbelliferyl-beta-D-glucuronide function as a β-glucuronidase substrate in microbial detection?
MUG is hydrolyzed by β-glucuronidase, an enzyme produced by Escherichia coli and other β-glucuronidase-positive organisms, releasing fluorescent 4-methylumbelliferone (4-MU). This fluorescence (excitation 365 nm, emission 445 nm) enables rapid, sensitive detection of enzymatic activity. MUG is incorporated into selective media (e.g., EC-MUG broth) to distinguish β-glucuronidase-positive E. coli from other coliforms. Methodologically, fluorescence intensity should be quantified using a fluorimeter, and results validated against negative controls to avoid false positives from autofluorescence or abiotic hydrolysis .
Advanced Question: How should researchers design experiments to detect low-abundance E. coli in complex environmental or food matrices using MUG?
Optimize sample pretreatment to reduce background fluorescence:
- Pre-filtration (0.45 µm) to remove particulate matter interfering with fluorimetric readings .
- Use selective enrichment media (e.g., lauryl tryptose broth with MUG) to suppress non-target microbes.
- Incubate samples at 44.5°C for 18–24 hours to favor E. coli growth while inhibiting mesophilic competitors .
- Quantify β-glucuronidase activity via fluorescence thresholding (e.g., >50 relative fluorescence units) to distinguish true positives from background noise .
Basic Question: What are the critical controls required when using MUG in β-glucuronidase activity assays?
- Negative control : Use β-glucuronidase-deficient strains (e.g., Klebsiella pneumoniae) to confirm substrate specificity .
- Substrate autofluorescence control : Include a sterile medium + MUG sample to detect non-enzymatic hydrolysis .
- Inhibition control : Spike samples with a known concentration of E. coli to assess matrix interference (e.g., acids in fruit juices suppress fluorescence) .
Advanced Question: How can researchers resolve contradictions in MUG-based assay performance across commercial preparations?
Discrepancies in sensitivity (e.g., false negatives in treated water samples) arise from variations in MUG purity, solubility, or stabilizers. To address this:
- Validate batches : Compare commercial MUG preparations against a standardized reference (e.g., Sigma-Aldrich 99% purity) using a E. coli type strain (ATCC 25922) .
- Optimize protocols : Adjust substrate concentration (e.g., 0.05–0.1 g/L in EC-MUG broth) and incubation time to account for lot-specific differences .
Advanced Question: What mechanisms underlie MUG’s role in hyaluronan (HA) synthesis inhibition, and how can this be leveraged in biomedical research?
MUG is metabolized to 4-MU, which inhibits HA synthesis by depleting UDP-glucuronic acid (HA precursor) and directly blocking HA synthase activity. For experimental applications:
- In vivo models : Administer MUG orally (100–200 mg/kg in mice) and monitor serum 4-MU:4-MUG equilibrium via LC–MS/MS to assess bioavailability .
- Tissue-specific effects : Use intravital microscopy to track 4-MU fluorescence in target organs (e.g., pancreas, liver) to correlate HA inhibition with therapeutic outcomes .
Basic Question: How is MUG applied in plant molecular biology for promoter activity assays?
In GUS reporter systems, MUG serves as a fluorogenic substrate to quantify β-glucuronidase activity driven by specific promoters. Methodologically:
- Homogenize plant tissues in extraction buffer (50 mM NaPO₄, pH 7.0).
- Incubate lysate with 1 mM MUG at 37°C for 1–4 hours.
- Terminate reactions with 0.2 M Na₂CO₃ and measure fluorescence. Normalize activity to total protein content (e.g., Bradford assay) .
Advanced Question: How can researchers optimize zymography protocols using MUG to detect β-glucosidase activity in fungal secretomes?
- Electrophoresis : Use 10% native PAGE to preserve enzyme activity.
- Substrate overlay : Post-electrophoresis, incubate gels in 5 µM MUG (pH 4.0 citrate-phosphate buffer) for 1 hour at 50°C.
- Visualization : Capture fluorescence under UV light (302 nm). Include a positive control (e.g., Trichoderma reesei cellulase) and quantify bands using densitometry .
Advanced Question: What strategies improve MUG-based assays in high-background environments (e.g., eutrophic water)?
- Dual-enzyme assays : Combine MUG with chromogenic substrates (e.g., ONPG for β-galactosidase) to differentiate E. coli from non-target β-glucuronidase producers .
- Flow cytometry : Couple fluorescence-activated cell sorting (FACS) with MUG hydrolysis to isolate live β-glucuronidase-positive cells .
Basic Question: What are the limitations of MUG in detecting atypical E. coli strains?
Some E. coli variants (e.g., O157:H7) lack β-glucuronidase activity. Confirm negative results with sorbitol fermentation tests or immunoassays (e.g., anti-O157 antibodies) .
Advanced Question: How does MUG hydrolysis kinetics vary across bacterial taxa, and what implications does this have for ecological studies?
- Kinetic profiling : Measure Vmax and Km for β-glucuronidase in environmental isolates (e.g., Citrobacter, Enterobacter) to assess substrate specificity.
- Ecological inference : Correlate hydrolysis rates with fecal pollution levels using regression models (e.g., MUG-HR vs. E. coli MPN in river water) .
属性
IUPAC Name |
(2S,3S,4S,5R,6S)-3,4,5-trihydroxy-6-(4-methyl-2-oxochromen-7-yl)oxyoxane-2-carboxylic acid | |
---|---|---|
Source | PubChem | |
URL | https://pubchem.ncbi.nlm.nih.gov | |
Description | Data deposited in or computed by PubChem | |
InChI |
InChI=1S/C16H16O9/c1-6-4-10(17)24-9-5-7(2-3-8(6)9)23-16-13(20)11(18)12(19)14(25-16)15(21)22/h2-5,11-14,16,18-20H,1H3,(H,21,22)/t11-,12-,13+,14-,16+/m0/s1 | |
Source | PubChem | |
URL | https://pubchem.ncbi.nlm.nih.gov | |
Description | Data deposited in or computed by PubChem | |
InChI Key |
ARQXEQLMMNGFDU-JHZZJYKESA-N | |
Source | PubChem | |
URL | https://pubchem.ncbi.nlm.nih.gov | |
Description | Data deposited in or computed by PubChem | |
Canonical SMILES |
CC1=CC(=O)OC2=C1C=CC(=C2)OC3C(C(C(C(O3)C(=O)O)O)O)O | |
Source | PubChem | |
URL | https://pubchem.ncbi.nlm.nih.gov | |
Description | Data deposited in or computed by PubChem | |
Isomeric SMILES |
CC1=CC(=O)OC2=C1C=CC(=C2)O[C@H]3[C@@H]([C@H]([C@@H]([C@H](O3)C(=O)O)O)O)O | |
Source | PubChem | |
URL | https://pubchem.ncbi.nlm.nih.gov | |
Description | Data deposited in or computed by PubChem | |
Molecular Formula |
C16H16O9 | |
Source | PubChem | |
URL | https://pubchem.ncbi.nlm.nih.gov | |
Description | Data deposited in or computed by PubChem | |
DSSTOX Substance ID |
DTXSID10891502 | |
Record name | 4-Methylumbelliferyl glucuronide | |
Source | EPA DSSTox | |
URL | https://comptox.epa.gov/dashboard/DTXSID10891502 | |
Description | DSSTox provides a high quality public chemistry resource for supporting improved predictive toxicology. | |
Molecular Weight |
352.29 g/mol | |
Source | PubChem | |
URL | https://pubchem.ncbi.nlm.nih.gov | |
Description | Data deposited in or computed by PubChem | |
CAS No. |
6160-80-1 | |
Record name | 4-Methylumbelliferyl β-D-glucuronide | |
Source | CAS Common Chemistry | |
URL | https://commonchemistry.cas.org/detail?cas_rn=6160-80-1 | |
Description | CAS Common Chemistry is an open community resource for accessing chemical information. Nearly 500,000 chemical substances from CAS REGISTRY cover areas of community interest, including common and frequently regulated chemicals, and those relevant to high school and undergraduate chemistry classes. This chemical information, curated by our expert scientists, is provided in alignment with our mission as a division of the American Chemical Society. | |
Explanation | The data from CAS Common Chemistry is provided under a CC-BY-NC 4.0 license, unless otherwise stated. | |
Record name | 4-Methylumbelliferyl glucuronide | |
Source | ChemIDplus | |
URL | https://pubchem.ncbi.nlm.nih.gov/substance/?source=chemidplus&sourceid=0006160801 | |
Description | ChemIDplus is a free, web search system that provides access to the structure and nomenclature authority files used for the identification of chemical substances cited in National Library of Medicine (NLM) databases, including the TOXNET system. | |
Record name | .beta.-D-Glucopyranosiduronic acid, 4-methyl-2-oxo-2H-1-benzopyran-7-yl | |
Source | EPA Chemicals under the TSCA | |
URL | https://www.epa.gov/chemicals-under-tsca | |
Description | EPA Chemicals under the Toxic Substances Control Act (TSCA) collection contains information on chemicals and their regulations under TSCA, including non-confidential content from the TSCA Chemical Substance Inventory and Chemical Data Reporting. | |
Record name | 4-Methylumbelliferyl glucuronide | |
Source | EPA DSSTox | |
URL | https://comptox.epa.gov/dashboard/DTXSID10891502 | |
Description | DSSTox provides a high quality public chemistry resource for supporting improved predictive toxicology. | |
Record name | 4-methyl-2-oxo-2H-1-benzopyran-7-yl β-D-glucopyranosiduronic acid | |
Source | European Chemicals Agency (ECHA) | |
URL | https://echa.europa.eu/substance-information/-/substanceinfo/100.025.624 | |
Description | The European Chemicals Agency (ECHA) is an agency of the European Union which is the driving force among regulatory authorities in implementing the EU's groundbreaking chemicals legislation for the benefit of human health and the environment as well as for innovation and competitiveness. | |
Explanation | Use of the information, documents and data from the ECHA website is subject to the terms and conditions of this Legal Notice, and subject to other binding limitations provided for under applicable law, the information, documents and data made available on the ECHA website may be reproduced, distributed and/or used, totally or in part, for non-commercial purposes provided that ECHA is acknowledged as the source: "Source: European Chemicals Agency, http://echa.europa.eu/". Such acknowledgement must be included in each copy of the material. ECHA permits and encourages organisations and individuals to create links to the ECHA website under the following cumulative conditions: Links can only be made to webpages that provide a link to the Legal Notice page. | |
Retrosynthesis Analysis
AI-Powered Synthesis Planning: Our tool employs the Template_relevance Pistachio, Template_relevance Bkms_metabolic, Template_relevance Pistachio_ringbreaker, Template_relevance Reaxys, Template_relevance Reaxys_biocatalysis model, leveraging a vast database of chemical reactions to predict feasible synthetic routes.
One-Step Synthesis Focus: Specifically designed for one-step synthesis, it provides concise and direct routes for your target compounds, streamlining the synthesis process.
Accurate Predictions: Utilizing the extensive PISTACHIO, BKMS_METABOLIC, PISTACHIO_RINGBREAKER, REAXYS, REAXYS_BIOCATALYSIS database, our tool offers high-accuracy predictions, reflecting the latest in chemical research and data.
Strategy Settings
Precursor scoring | Relevance Heuristic |
---|---|
Min. plausibility | 0.01 |
Model | Template_relevance |
Template Set | Pistachio/Bkms_metabolic/Pistachio_ringbreaker/Reaxys/Reaxys_biocatalysis |
Top-N result to add to graph | 6 |
Feasible Synthetic Routes
体外研究产品的免责声明和信息
请注意,BenchChem 上展示的所有文章和产品信息仅供信息参考。 BenchChem 上可购买的产品专为体外研究设计,这些研究在生物体外进行。体外研究,源自拉丁语 "in glass",涉及在受控实验室环境中使用细胞或组织进行的实验。重要的是要注意,这些产品没有被归类为药物或药品,他们没有得到 FDA 的批准,用于预防、治疗或治愈任何医疗状况、疾病或疾病。我们必须强调,将这些产品以任何形式引入人类或动物的身体都是法律严格禁止的。遵守这些指南对确保研究和实验的法律和道德标准的符合性至关重要。