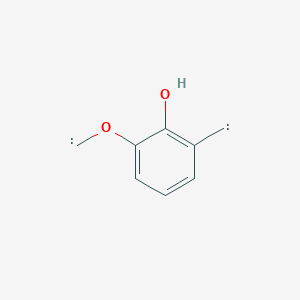
酚醛树脂
概述
描述
Phenol-formaldehyde resin is a synthetic polymer obtained by the reaction of phenol with formaldehyde. It was the first commercial synthetic resin and is commonly known for its use in Bakelite, a pioneering plastic. Phenol-formaldehyde resins are widely used in the production of molded products, coatings, adhesives, and as a basis for various industrial applications .
科学研究应用
Phenol-formaldehyde resins have a wide range of scientific research applications:
Biology: Utilized in laboratory equipment and as a component in various biological assays.
Medicine: Employed in medical devices and as a component in certain drug delivery systems.
生化分析
Biochemical Properties
Phenol-formaldehyde resin is a high-performance and very stable thermosetting polymer with high mechanical properties and high temperature and chemical resistance . It is produced by the reaction of phenol (hydroxyl benzene) and formaldehyde
Cellular Effects
Phenol-formaldehyde resin has been found to have significant effects on various types of cells and cellular processes. For example, it has been shown to increase the cellular reactive oxygen species (ROS) and reduce the cell viability of human lung epithelial adenocarcinoma cells (A549)
Molecular Mechanism
The molecular mechanism of action of phenol-formaldehyde resin involves its reaction with phenol to form a network polymer . This involves the formation of a hydroxymethyl phenol, which can react with another free ortho or para site, or with another hydroxymethyl group . The first reaction gives a methylene bridge, and the second forms an ether bridge .
Temporal Effects in Laboratory Settings
It is known that the resin has excellent stability and does not degrade easily .
Transport and Distribution
Phenol-formaldehyde resin is known to diffuse effectively into the cell wall, as shown in studies involving wood cell walls
准备方法
Phenol-formaldehyde resins are synthesized through a step-growth polymerization reaction that can be either acid- or base-catalyzed. There are two primary methods for industrial production:
化学反应分析
Phenol-formaldehyde resins undergo various chemical reactions, including:
Condensation Reactions: Phenol reacts with formaldehyde at the ortho and para sites, forming hydroxymethyl phenol.
Oxidation and Reduction: These reactions are less common but can occur under specific conditions, altering the resin’s properties.
Substitution Reactions: Phenolic hydroxyl groups can undergo substitution reactions, introducing new functional groups to modify the resin’s properties.
Common reagents include sulfuric acid, oxalic acid, hydrochloric acid, and various base catalysts. Major products include bisphenol F and higher phenol oligomers .
作用机制
The mechanism of action of phenol-formaldehyde resins involves the formation of a highly crosslinked polymer network through condensation reactions. The hydroxymethyl groups react with free ortho or para sites on the phenol ring, forming methylene or ether bridges. This results in a rigid, thermosetting polymer with high mechanical strength and chemical resistance .
相似化合物的比较
Phenol-formaldehyde resins are compared with other similar compounds such as:
Urea-Formaldehyde Resins: These resins are also used as adhesives but have lower moisture resistance compared to phenol-formaldehyde resins.
Melamine-Formaldehyde Resins: Known for their high resistance to heat and chemicals, making them suitable for high-performance applications.
Phenol-formaldehyde resins are unique due to their high mechanical strength, chemical resistance, and cost-effectiveness, making them suitable for a wide range of applications .
生物活性
Phenol-formaldehyde resin (PF resin) is a widely used thermosetting polymer known for its excellent adhesive properties, durability, and resistance to heat and chemicals. However, its biological activity raises concerns regarding toxicity, allergenicity, and potential health effects on humans and the environment. This article explores the biological activity of PF resin, focusing on its health impacts, mechanisms of toxicity, and recent research findings.
Overview of Phenol-Formaldehyde Resin
PF resin is created through the polymerization of phenol and formaldehyde, typically in an alkaline environment. It is extensively used in the production of wood-based panels, adhesives, and coatings due to its strong bonding capabilities and thermal stability. Despite its industrial benefits, PF resin poses several health risks that necessitate thorough investigation.
Allergic Reactions and Dermatitis
Epidemiological studies indicate that PF resins can cause contact dermatitis in a subset of individuals. Approximately 1-3% of people with skin diseases show positive reactions to PF resins, suggesting a significant allergenic potential . In a multicenter patch testing study involving 2,504 patients, 27 individuals (1.1%) reacted positively to a specific PF resin variant (PFR-2), highlighting the importance of monitoring allergic responses in occupational settings .
Respiratory Effects
Exposure to PF resin fumes has been associated with chronic airway diseases. A study involving workers in a filter-manufacturing plant revealed that those exposed for over five years exhibited decreased pulmonary function (measured by FEV1.0/FVC ratios) compared to non-exposed individuals . Symptoms such as chronic cough and sputum production were also reported among exposed workers.
Genotoxicity and Reproductive Effects
The genotoxic potential of PF resins remains equivocal. Some studies have reported chromosomal aberrations in workers exposed to PF resins, while others have shown no significant mutagenic effects . Limited data suggest potential reproductive toxicity; for instance, damage to rat spermatocytes has been noted following inhalation exposure .
Mechanisms of Toxicity
PF resin's biological activity can be attributed to several mechanisms:
- Irritation : PF resins are known to irritate the skin, eyes, and mucosal membranes upon contact or inhalation .
- Sensitization : The presence of sensitizing agents within PF resins can lead to allergic reactions upon repeated exposure.
- Volatile Organic Compounds (VOCs) : The release of formaldehyde—a known carcinogen—during the curing process poses additional health risks .
Recent Research Findings
Recent studies have focused on modifying PF resins to enhance their properties while reducing toxicity:
- Bio-Oil Modified Resins : Research indicates that incorporating bio-oil into PF resins can improve their bonding strength and aging resistance while potentially lowering costs . The bio-oil components positively affected the resin's microstructure and performance characteristics.
Modification | Effect on Bonding Strength | Aging Resistance |
---|---|---|
Bio-Oil Addition | Improved | Enhanced |
Phenol Substitution | Reduced | Variable |
- Mannich Bases Resins : A novel approach involved synthesizing PF resins using Mannich bases derived from primary amines. These resins exhibited promising biological activity profiles and could serve as safer alternatives in various applications .
Case Studies
- Plywood Manufacturing Exposure Assessment :
- Long-Term Health Monitoring :
属性
InChI |
InChI=1S/C8H6O2/c1-6-4-3-5-7(10-2)8(6)9/h1-5,9H | |
---|---|---|
Source | PubChem | |
URL | https://pubchem.ncbi.nlm.nih.gov | |
Description | Data deposited in or computed by PubChem | |
InChI Key |
KXGFMDJXCMQABM-UHFFFAOYSA-N | |
Source | PubChem | |
URL | https://pubchem.ncbi.nlm.nih.gov | |
Description | Data deposited in or computed by PubChem | |
Canonical SMILES |
[CH]C1=C(C(=CC=C1)O[CH])O | |
Source | PubChem | |
URL | https://pubchem.ncbi.nlm.nih.gov | |
Description | Data deposited in or computed by PubChem | |
Molecular Formula |
C8H6O2 | |
Source | PubChem | |
URL | https://pubchem.ncbi.nlm.nih.gov | |
Description | Data deposited in or computed by PubChem | |
Molecular Weight |
134.13 g/mol | |
Source | PubChem | |
URL | https://pubchem.ncbi.nlm.nih.gov | |
Description | Data deposited in or computed by PubChem | |
CAS No. |
9003-35-4 | |
Record name | Phenol-formaldehyde resin | |
Source | ChemIDplus | |
URL | https://pubchem.ncbi.nlm.nih.gov/substance/?source=chemidplus&sourceid=0009003354 | |
Description | ChemIDplus is a free, web search system that provides access to the structure and nomenclature authority files used for the identification of chemical substances cited in National Library of Medicine (NLM) databases, including the TOXNET system. | |
Retrosynthesis Analysis
AI-Powered Synthesis Planning: Our tool employs the Template_relevance Pistachio, Template_relevance Bkms_metabolic, Template_relevance Pistachio_ringbreaker, Template_relevance Reaxys, Template_relevance Reaxys_biocatalysis model, leveraging a vast database of chemical reactions to predict feasible synthetic routes.
One-Step Synthesis Focus: Specifically designed for one-step synthesis, it provides concise and direct routes for your target compounds, streamlining the synthesis process.
Accurate Predictions: Utilizing the extensive PISTACHIO, BKMS_METABOLIC, PISTACHIO_RINGBREAKER, REAXYS, REAXYS_BIOCATALYSIS database, our tool offers high-accuracy predictions, reflecting the latest in chemical research and data.
Strategy Settings
Precursor scoring | Relevance Heuristic |
---|---|
Min. plausibility | 0.01 |
Model | Template_relevance |
Template Set | Pistachio/Bkms_metabolic/Pistachio_ringbreaker/Reaxys/Reaxys_biocatalysis |
Top-N result to add to graph | 6 |
Feasible Synthetic Routes
Q1: What is the molecular formula of Phenol-Formaldehyde Resin?
A1: Phenol-Formaldehyde resins don't have a single, well-defined molecular formula. They are complex mixtures of oligomers with varying chain lengths and degrees of crosslinking. The structure is formed through the polymerization of phenol (C6H5OH) and formaldehyde (CH2O).
Q2: What is the typical molecular weight of Phenol-Formaldehyde Resin?
A2: Similar to the molecular formula, the molecular weight of PF resins is not fixed and depends on the synthesis conditions and degree of polymerization. The weight can range from a few hundred to several thousand grams per mole.
Q3: How can you determine the structure of Phenol-Formaldehyde Resin?
A3: Several spectroscopic techniques are used to characterize the structure of PF resins. These include:
- Fourier Transform Infrared Spectroscopy (FTIR): Identifies functional groups like -OH, -CH2-, and aromatic rings. [, , , , , ]
- Nuclear Magnetic Resonance (NMR): Provides information on the types and arrangements of hydrogen and carbon atoms within the resin structure. [, , ]
- Size-Exclusion Chromatography (SEC): Helps determine the molecular weight distribution of the resin. []
Q4: Are there different types of Phenol-Formaldehyde Resins?
A4: Yes, PF resins can be broadly categorized into two main types based on the reaction conditions and formaldehyde/phenol ratio:
- Novolac Resins: Synthesized with an acidic catalyst and a formaldehyde to phenol ratio less than 1. These resins require a curing agent, typically hexamethylenetetramine, to form a crosslinked network. [, , , ]
- Resol Resins: Synthesized with a basic catalyst and a formaldehyde to phenol ratio greater than 1. These resins are self-curing upon heating. [, , , , ]
Q5: How does the formaldehyde/phenol ratio affect the resin properties?
A5: The formaldehyde/phenol ratio significantly impacts the properties of the final resin. Higher ratios generally lead to increased crosslinking density, resulting in harder, more brittle, and more thermally stable resins. [, , ]
Q6: Can the properties of Phenol-Formaldehyde Resin be modified?
A6: Yes, PF resins can be modified to tailor their properties for specific applications. This can be achieved through various methods:
- Co-polymerization: Incorporating other monomers during synthesis, such as lignin, to enhance specific properties like biodegradability or reduce cost. [, , , , ]
- Blending: Mixing PF resin with other polymers, like epoxy resins or polyvinylpyrrolidone, to improve flexibility, toughness, or adhesion. [, , , , ]
- Addition of Additives: Incorporating fillers, plasticizers, or reinforcing agents to modify properties such as strength, thermal stability, or flame retardancy. [, , , , , ]
Q7: What are the key properties of Phenol-Formaldehyde Resins that make them suitable for various applications?
A7: PF resins possess a combination of desirable properties, including:
Q8: What are some common applications of Phenol-Formaldehyde Resins?
A8: PF resins are used in a wide range of applications, including:
- Wood adhesives: For plywood, oriented strand board (OSB), and other wood-based composites. [, , , ]
- Molding compounds: For electrical components, automotive parts, and household appliances. [, ]
- Coatings: For corrosion protection, insulation, and decorative finishes. [, ]
Q9: How does the curing process affect the properties of Phenol-Formaldehyde Resin?
A9: Curing transforms the liquid resin into a rigid, crosslinked network, significantly influencing its final properties. Factors like curing temperature, time, and the presence of catalysts or hardeners affect the degree of crosslinking and ultimately the strength, thermal stability, and chemical resistance of the cured resin. [, , , ]
Q10: What are the limitations of Phenol-Formaldehyde Resins?
A10: Some limitations of PF resins include:
- Formaldehyde emission: Formaldehyde release from PF resins, particularly during curing and in the early stages of product life, raises environmental and health concerns. [, , ]
- Dark color: The inherent dark color of PF resins can limit their use in applications requiring light color or transparency. []
Q11: What are the environmental concerns associated with Phenol-Formaldehyde Resins?
A11: The primary environmental concern is the release of formaldehyde, a volatile organic compound (VOC) classified as a human carcinogen. Formaldehyde emission occurs during resin production, curing, and from the final product. [, , ]
Q12: Are there any regulations regarding the use and disposal of Phenol-Formaldehyde Resins?
A12: Yes, various regulations and standards address formaldehyde emissions from PF resin-containing products, such as wood-based composites. These regulations vary by country and application, focusing on limiting formaldehyde release to protect human health and the environment. [, ]
Q13: What are some strategies to mitigate the environmental impact of Phenol-Formaldehyde Resins?
A13: Researchers are exploring several strategies to reduce formaldehyde emissions and the environmental footprint of PF resins:
- Developing low-formaldehyde or formaldehyde-free resins [, ]
- Exploring bio-based alternatives to phenol, like lignin [, , ]
Q14: What are some areas of ongoing research in Phenol-Formaldehyde Resin technology?
A14: Research on PF resins continues to focus on:
- Developing sustainable and bio-based alternatives [, , , ]
- Reducing or eliminating formaldehyde emissions [, , ]
- Improving mechanical properties like toughness and impact resistance [, , ]
Q15: What are some promising alternatives to Phenol-Formaldehyde Resins?
A15: Researchers are investigating several alternatives to PF resins, including:
体外研究产品的免责声明和信息
请注意,BenchChem 上展示的所有文章和产品信息仅供信息参考。 BenchChem 上可购买的产品专为体外研究设计,这些研究在生物体外进行。体外研究,源自拉丁语 "in glass",涉及在受控实验室环境中使用细胞或组织进行的实验。重要的是要注意,这些产品没有被归类为药物或药品,他们没有得到 FDA 的批准,用于预防、治疗或治愈任何医疗状况、疾病或疾病。我们必须强调,将这些产品以任何形式引入人类或动物的身体都是法律严格禁止的。遵守这些指南对确保研究和实验的法律和道德标准的符合性至关重要。