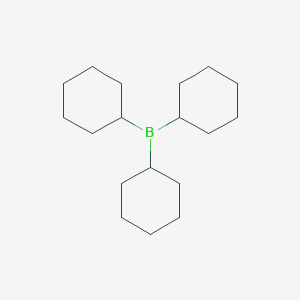
Tricyclohexylborane
概述
描述
Tricyclohexylborane (C₆H₁₁)₃B is a trialkylborane synthesized via hydroboration of cyclohexene with borane-dimethyl sulfide (BH₃·SMe₂). This reaction typically requires three equivalents of cyclohexene per equivalent of BH₃, yielding a tri-substituted borane. The compound is notable for its steric bulk due to three cyclohexyl groups, which significantly influences its reactivity and physical properties. This compound is soluble in ethereal solvents and is primarily used in hydroboration-oxidation reactions to produce cyclohexanol .
准备方法
Synthetic Routes and Reaction Conditions: Tricyclohexylborane is typically synthesized through the hydroboration of cyclohexene with borane (BH₃). The reaction proceeds as follows: [ \text{3 C₆H₁₀ + BH₃ → B(C₆H₁₁)₃} ] This reaction is carried out in an inert solvent such as tetrahydrofuran (THF) under anhydrous conditions to prevent the hydrolysis of borane. The reaction is exothermic and requires careful control of temperature to avoid side reactions.
Industrial Production Methods: Industrial production of this compound follows a similar synthetic route but on a larger scale. The process involves the continuous addition of cyclohexene to a borane solution under controlled conditions to ensure complete conversion and high yield. The product is then purified through distillation or crystallization.
化学反应分析
Hydroboration Reactions
Tricyclohexylborane participates in hydroboration, where boron adds across carbon-carbon double bonds. Key features include:
-
Anti-Markovnikov Selectivity : Like other trialkylboranes, it follows anti-Markovnikov addition due to boron’s electrophilic character .
-
Steric Effects : The bulky cyclohexyl groups hinder reactivity with sterically crowded alkenes, favoring substrates with accessible π-bonds .
-
Stepwise Addition : Each BH₃ equivalent adds sequentially, forming mono-, di-, and tri-alkylboranes. For this compound, full conversion requires excess alkene12.
Example Reaction :
Oxidation to Alcohols
This compound undergoes oxidation to yield alcohols via hydroboration-oxidation:
-
Conditions : Treatment with hydrogen peroxide (H₂O₂) and aqueous NaOH .
-
Stereospecificity : The reaction retains the stereochemistry of the hydroboration step, producing syn-diols from cyclic intermediates12.
-
Yield : Oxidation efficiency depends on steric accessibility; bulky substrates may require prolonged reaction times .
Reaction Pathway :
Carbonylation and Homologation
This compound reacts with carbon monoxide (CO) to form homologated products:
-
Mechanism : CO inserts into the B–C bond, followed by alkyl migration to the carbonyl carbon .
-
Products : Primary alcohols after reduction (e.g., with NaBH₄) .
Example :
Lewis Acid Catalysis
While less acidic than tris(pentafluorophenyl)borane (BCF), this compound can act as a Lewis acid in:
-
Hydrosilylation : Activates Si–H bonds for reduction of carbonyls .
-
Polymerization : Initiates cationic polymerization of alkenes, though steric bulk limits efficiency compared to BCF .
Comparative Activity :
Property | This compound | BCF |
---|---|---|
Lewis Acidity (Gutmann) | Low | High |
Steric Bulk | High | Moderate |
Catalytic Applications | Limited by steric hindrance | Broad (e.g., FLP chemistry) |
Transmetallation and Cross-Coupling
This compound transfers cyclohexyl groups in transmetallation reactions:
-
Grignard Exchange : Reacts with Grignard reagents (RMgX) to form mixed alkylboranes .
-
Suzuki-Miyaura : Limited utility due to slow transmetallation kinetics with aryl halides .
Limitation : Bulky cyclohexyl groups reduce compatibility with catalytic systems optimized for smaller boranes .
Stability and Decomposition
科学研究应用
Hydroboration Reactions
Hydroboration of Alkenes and Alkynes
TCHB is used extensively in hydroboration reactions, where it reacts with alkenes and alkynes to form organoboranes. This process enables the regioselective functionalization of olefins, leading to the formation of alcohols after oxidation. The hydroboration reaction is crucial for synthesizing various organic compounds due to its mild conditions and high selectivity .
Case Study: Hydroboration of Cyclic Dienes
A systematic study demonstrated the hydroboration of 1,3-cyclohexadiene using TCHB, leading to the formation of new boron-containing polymeric materials. The reaction conditions favored dihydroboration, resulting in a polymeric network stabilized by B–H–B bridges. Solid-state NMR analysis confirmed the presence of tricyclohexylborane within these materials, indicating its potential as a moisture scavenger .
Synthesis of Boron-Containing Materials
Polymeric Boron Compounds
TCHB has been utilized in synthesizing tunable boron-containing hydrocarbon polymeric materials through a cascade hydroboration process. These materials exhibit unique properties that can be tailored for specific applications, such as instant moisture absorption and enhanced mechanical properties .
Material Type | Synthesis Method | Key Properties |
---|---|---|
Polymeric Boron Compounds | Hydroboration Cascade | Moisture scavenging, tunable mechanical properties |
Organoboranes | Hydroboration of Alkenes | High regioselectivity, mild reaction conditions |
Catalytic Applications
Frustrated Lewis Pair (FLP) Systems
TCHB has been explored in FLP systems, where it acts as a Lewis acid in combination with Lewis bases to activate small molecules such as hydrogen. This application is particularly relevant in hydrogenation reactions and the activation of inert bonds, showcasing TCHB's versatility in catalysis .
Reactions with Carbon Dioxide
Carbon Dioxide Activation
Recent studies have investigated the reaction of TCHB with carbon dioxide in diglyme, leading to the formation of cyclic glycol esters. This reaction highlights TCHB's potential role in CO2 utilization strategies and carbon capture technologies .
Metallation Reactions
Metallation with Transition Metals
TCHB can participate in metallation reactions, where it reacts with transition metal complexes to form organometallic species. These reactions are vital for developing new catalysts and materials with enhanced functionalities .
作用机制
The mechanism of action of tricyclohexylborane in hydroboration involves the addition of the boron-hydrogen bond across the carbon-carbon double bond of an alkene. This reaction occurs in a concerted manner, where the boron atom attaches to the less substituted carbon, and the hydrogen atom attaches to the more substituted carbon. This results in syn addition, where both the boron and hydrogen atoms add to the same side of the double bond.
相似化合物的比较
Structural and Spectroscopic Comparisons
Dicyclohexylborane (C₆H₁₁)₂BH
- Synthesis : Forms as the kinetic product during rapid hydroboration of cyclohexene. It features a B–H–B bridged structure (R₂BH)₂, which is stabilized by B–H–B interactions .
- ¹¹B NMR : Exhibits a narrow peak at +29 ppm (solution) due to reduced quadrupolar coupling (2.7 MHz) and lower chemical shift anisotropy compared to tricyclohexylborane .
- Reactivity : Acts as a precursor to insoluble borane polymers when reacted with dienes, forming crosslinked networks via B–H–B bridges .
This compound (C₆H₁₁)₃B
- ¹¹B NMR : Shows a broad resonance at +82 ppm (solution) with high quadrupolar coupling (5 MHz) and chemical shift anisotropy (~90 ppm), reflecting its anisotropic trigonal planar geometry .
- Thermodynamic Stability : Forms as the thermodynamic product from dicyclohexylborane under prolonged reaction conditions, despite a high energy barrier (20.4 kcal/mol) due to steric hindrance .
Table 1: Key Spectroscopic and Structural Properties
Compound | ¹¹B NMR Shift (ppm) | Quadrupolar Coupling | Chemical Shift Anisotropy | Structure |
---|---|---|---|---|
This compound | +82 | 5 MHz | ~90 ppm | Trigonal planar |
Dicyclohexylborane | +29 | 2.7 MHz | Minimal | B–H–B bridged dimer |
Reactivity in Cross-Coupling Reactions
- Only one cyclohexyl group transfers per boron center, yielding moderate product yields. This inertness is attributed to steric hindrance and the reluctance of secondary alkyl groups to undergo transmetalation .
- Triethylborane (Et₃B) : A primary alkylborane, it reacts efficiently in cross-couplings due to lower steric bulk and faster transmetalation kinetics .
- Triphenylborane (Ph₃B) : Used as a catalyst in polymerization and hydrocyanation reactions, leveraging its aromatic substituents for π-orbital interactions .
Table 2: Cross-Coupling Reactivity
Compound | Alkyl Type | Reactivity with Aryl Chlorides | Key Application |
---|---|---|---|
This compound | Secondary | Low (moderate yields) | Hydroboration-oxidation |
Triethylborane | Primary | High | Radical initiation, Couplings |
Triphenylborane | Aryl | N/A | Polymerization catalysis |
Autoxidation Behavior
This compound undergoes autoxidation with an induction period influenced by steric effects. It is less reactive than tri(primary alkyl)boranes (e.g., Et₃B) but more reactive than tri(isobutyl)borane (Bus₃B). The mechanism likely involves radical intermediates formed via O₂ adducts, though the exact pathway remains unresolved .
常见问题
Basic Research Questions
Q. What are the standard synthetic procedures for tricyclohexylborane, and how can experimental parameters be optimized for yield and purity?
this compound is synthesized via hydroboration of cyclohexene using borane (BH₃) in solvents like tetrahydrofuran (THF) or diethyl ether. A stoichiometric ratio of 3:1 (cyclohexene: BH₃) ensures complete conversion to the trialkylborane product . Isolation involves filtering insoluble dicyclohexylborane (kinetic product) and concentrating the filtrate to recover this compound (thermodynamic product). Yield optimization requires controlled reagent addition rates and reaction times to favor this compound formation, as rapid borane addition promotes bridged dicyclohexylborane intermediates . Purity is confirmed via recrystallization and spectroscopic analysis (e.g., ¹¹B NMR at +81 ppm for this compound) .
Q. How is this compound characterized using spectroscopic methods?
Key characterization techniques include:
- FT-IR : Peaks for terminal B–Ht (2600–2500 cm⁻¹), bridged B–Hb (1600–1500 cm⁻¹), and B–C bonds (1200–800 cm⁻¹). Absence of C=C stretches (1800–1700 cm⁻¹) confirms complete hydroboration .
- ¹¹B NMR : this compound exhibits a distinct signal at +81 ppm in solution, whereas bridged dicyclohexylborane appears at +29 ppm .
- Solid-state NMR : Differentiates between B–H–B bridged species and unbridged boranes, resolving ambiguities in product identity .
Advanced Research Questions
Q. What factors influence the product distribution between dicyclohexylborane and this compound in hydroboration reactions?
Product distribution is governed by kinetic vs. thermodynamic control. Rapid borane addition favors dicyclohexylborane (R₂BH)₂, a kinetic product stabilized by B–H–B bridging. Prolonged reaction times or excess cyclohexene drive conversion to this compound (thermodynamic product) . Solvent choice (e.g., diethyl ether vs. diglyme) also modulates solubility and reaction pathways. DFT calculations (B3LYP/6-311++G) reveal a high activation barrier (~20.4 kcal mol⁻¹) for the third hydroboration step due to steric hindrance, explaining the slow formation of this compound .
Q. How can computational methods like DFT elucidate reaction pathways and steric effects in this compound synthesis?
Density functional theory (DFT) simulations at the B3LYP/6-311++G level quantify steric barriers during cyclohexene addition to borane intermediates. For example, the third hydroboration step exhibits a 20.4 kcal mol⁻¹ barrier, attributed to crowding from cyclohexyl substituents . These models predict regioselectivity and explain experimental observations, such as preferential formation of bridged intermediates. Computational data should be cross-validated with experimental kinetic studies and spectroscopic evidence (e.g., ¹¹B NMR) to resolve discrepancies .
Q. What are the common contradictions in reported data on this compound's reactivity, and how can they be resolved methodologically?
Discrepancies often arise from solvent effects, incomplete hydroboration, or misassignment of spectroscopic signals. For example, FT-IR alone cannot distinguish between R₂BHBH₃ and unbridged R₂BH species, necessitating complementary solid-state NMR analysis . To resolve contradictions, researchers should:
- Replicate experiments under strictly controlled conditions (e.g., anaerobic synthesis to prevent oxidation).
- Use isotopic labeling (e.g., deuterated solvents) to track reaction pathways.
- Compare computational predictions (e.g., DFT-derived vibrational frequencies) with experimental spectra .
Q. Methodological Guidance for Addressing Research Gaps
Q. How can researchers design experiments to probe the steric and electronic effects of this compound in catalytic applications?
Systematic studies should vary substituents on borane analogs (e.g., replacing cyclohexyl with smaller/larger groups) and monitor reactivity changes using kinetic assays and spectroscopic profiling. For electronic effects, employ Hammett parameters or computational electrostatic potential maps to correlate substituent effects with reaction rates .
Q. What strategies ensure reproducibility in tricycloclohexylborane synthesis and characterization?
属性
IUPAC Name |
tricyclohexylborane | |
---|---|---|
Source | PubChem | |
URL | https://pubchem.ncbi.nlm.nih.gov | |
Description | Data deposited in or computed by PubChem | |
InChI |
InChI=1S/C18H33B/c1-4-10-16(11-5-1)19(17-12-6-2-7-13-17)18-14-8-3-9-15-18/h16-18H,1-15H2 | |
Source | PubChem | |
URL | https://pubchem.ncbi.nlm.nih.gov | |
Description | Data deposited in or computed by PubChem | |
InChI Key |
SJXLNIDKIYXLBL-UHFFFAOYSA-N | |
Source | PubChem | |
URL | https://pubchem.ncbi.nlm.nih.gov | |
Description | Data deposited in or computed by PubChem | |
Canonical SMILES |
B(C1CCCCC1)(C2CCCCC2)C3CCCCC3 | |
Source | PubChem | |
URL | https://pubchem.ncbi.nlm.nih.gov | |
Description | Data deposited in or computed by PubChem | |
Molecular Formula |
C18H33B | |
Source | PubChem | |
URL | https://pubchem.ncbi.nlm.nih.gov | |
Description | Data deposited in or computed by PubChem | |
Molecular Weight |
260.3 g/mol | |
Source | PubChem | |
URL | https://pubchem.ncbi.nlm.nih.gov | |
Description | Data deposited in or computed by PubChem | |
Synthesis routes and methods
Procedure details
Retrosynthesis Analysis
AI-Powered Synthesis Planning: Our tool employs the Template_relevance Pistachio, Template_relevance Bkms_metabolic, Template_relevance Pistachio_ringbreaker, Template_relevance Reaxys, Template_relevance Reaxys_biocatalysis model, leveraging a vast database of chemical reactions to predict feasible synthetic routes.
One-Step Synthesis Focus: Specifically designed for one-step synthesis, it provides concise and direct routes for your target compounds, streamlining the synthesis process.
Accurate Predictions: Utilizing the extensive PISTACHIO, BKMS_METABOLIC, PISTACHIO_RINGBREAKER, REAXYS, REAXYS_BIOCATALYSIS database, our tool offers high-accuracy predictions, reflecting the latest in chemical research and data.
Strategy Settings
Precursor scoring | Relevance Heuristic |
---|---|
Min. plausibility | 0.01 |
Model | Template_relevance |
Template Set | Pistachio/Bkms_metabolic/Pistachio_ringbreaker/Reaxys/Reaxys_biocatalysis |
Top-N result to add to graph | 6 |
Feasible Synthetic Routes
体外研究产品的免责声明和信息
请注意,BenchChem 上展示的所有文章和产品信息仅供信息参考。 BenchChem 上可购买的产品专为体外研究设计,这些研究在生物体外进行。体外研究,源自拉丁语 "in glass",涉及在受控实验室环境中使用细胞或组织进行的实验。重要的是要注意,这些产品没有被归类为药物或药品,他们没有得到 FDA 的批准,用于预防、治疗或治愈任何医疗状况、疾病或疾病。我们必须强调,将这些产品以任何形式引入人类或动物的身体都是法律严格禁止的。遵守这些指南对确保研究和实验的法律和道德标准的符合性至关重要。