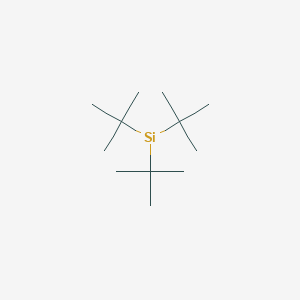
Tritert-butylsilicon
Overview
Description
Tritert-butylsilicon, more accurately identified as tritert-butyl(fluoro)silane (C₁₂H₂₇FSi), is a sterically hindered organosilicon compound featuring three tert-butyl substituents and one fluorine atom bonded to a central silicon atom . This compound is referenced in studies focusing on synthetic methodologies and spectroscopic characterization, though its applications remain less documented compared to other organosilicon derivatives .
Preparation Methods
The synthesis of tritert-butylsilicon typically involves the reaction of triethylsilane with tert-butyl alkyl lithium (t-BuLi) at low temperatures . This reaction is carried out under an inert atmosphere to prevent the compound from reacting with oxygen or moisture. The general reaction scheme is as follows:
[ \text{(CH}_3\text{CH}_2\text{)}_3\text{SiH} + 3 \text{t-BuLi} \rightarrow \text{(t-Bu)}_3\text{SiH} + 3 \text{LiCH}_2\text{CH}_3 ]
In industrial settings, the production of this compound may involve more scalable methods, but the fundamental reaction principles remain the same. The compound is typically purified through distillation or recrystallization to achieve the desired purity levels.
Chemical Reactions Analysis
Tritert-butylsilicon undergoes various chemical reactions, including:
Oxidation: The compound can be oxidized to form silanols or siloxanes. Common oxidizing agents include hydrogen peroxide and ozone.
Reduction: Reduction reactions can convert this compound to simpler silanes. Reducing agents such as lithium aluminum hydride (LiAlH4) are commonly used.
Substitution: this compound can participate in substitution reactions where the tert-butyl groups are replaced by other functional groups. Halogenation reactions using reagents like chlorine or bromine are typical examples.
The major products formed from these reactions depend on the specific reagents and conditions used. For instance, oxidation with hydrogen peroxide may yield silanols, while halogenation with chlorine can produce chlorosilanes.
Scientific Research Applications
Chemical Synthesis
Tritert-butylsilicon is primarily utilized in chemical synthesis due to its ability to serve as a precursor for various silicon-containing compounds. The synthesis of this compound typically involves the reaction of tert-butyl chloride with silicon-containing reagents. This method allows for the adjustment of its properties to suit specific applications.
Synthesis Method:
- Reactants: Tert-butyl chloride and silicon reagents
- Conditions: Varies based on the desired product characteristics
This versatility in synthesis is crucial for developing new materials and chemical processes.
Reactivity Studies
The reactivity of this compound has been extensively studied, revealing significant insights into its interactions with other chemical species. The compound's steric hindrance leads to unique reactivity patterns compared to less hindered silanes.
Key Findings:
- This compound exhibits lower reactivity than smaller silanes due to steric effects.
- It can participate in various reactions, including hydrosilylation and dehydrogenative coupling.
Understanding these interactions is essential for optimizing reaction conditions and developing new synthetic methodologies.
Material Science Applications
This compound plays a significant role in material science, particularly in the development of advanced materials with tailored properties. Its unique structure allows it to be incorporated into various materials, enhancing their performance.
Applications Include:
- Silicon-Based Nanomaterials: this compound can be used as a precursor for synthesizing silicon nanoparticles, which are valuable in electronics and energy storage.
- Coatings and Adhesives: The compound's properties make it suitable for formulating high-performance coatings that require chemical stability and resistance to degradation.
Case Studies
Several studies have highlighted the applications of this compound in different contexts:
These case studies demonstrate the diverse applications of this compound across various scientific disciplines.
Mechanism of Action
The mechanism of action of tritert-butylsilicon is influenced by the steric effects of the tert-butyl groups. These bulky groups create a significant steric hindrance, affecting the compound’s reactivity and interactions with other molecules. In glycosylation reactions, for example, this compound compounds can act as catalysts, facilitating the formation of glycosidic bonds through a single hydrogen bond mechanism.
Comparison with Similar Compounds
Comparison with Similar Compounds
Structural and Molecular Comparisons
The tert-butyl groups in tritert-butyl(fluoro)silane confer exceptional steric hindrance, distinguishing it from smaller trialkylsilanes (e.g., trimethyl- or triethylsilane) and silyl ethers (e.g., tert-butyldimethylsilyl derivatives). Key structural differences include:
The tert-butyl groups in tritert-butyl(fluoro)silane create a shielded silicon center, reducing nucleophilic attack compared to less bulky compounds like tetraethyl silicate, which hydrolyzes readily due to accessible ethoxy groups .
Spectroscopic Properties
- tert-Butyldimethylsilylglyoxylate: Shows IR peaks at 1716 cm⁻¹ (C=O stretch) and 1252 cm⁻¹ (Si-C), with ¹H NMR signals for tert-butyl groups at 1.05 ppm . tert-Butyldimethylsilyl triflate: Characteristic ¹H NMR tert-butyl signals at 0.95 ppm and a triflate CF₃ group at -78 ppm in ¹⁹F NMR .
The absence of electron-withdrawing groups (e.g., triflate) in tritert-butyl(fluoro)silane likely results in less deshielded silicon compared to silyl triflates .
Reactivity and Stability
- Hydrolysis Resistance :
Tritert-butyl(fluoro)silane’s bulky tert-butyl groups impede hydrolysis, unlike tetraethyl silicate, which rapidly reacts with water to form silicic acid . - Lewis Acidity :
While tert-butyldimethylsilyl triflate acts as a potent Lewis acid for silylation, tritert-butyl(fluoro)silane’s steric hindrance may limit its efficacy in similar reactions . - Thermal Stability: High steric bulk enhances thermal stability, a trait shared with tri-tert-butylphosphine (noted in unrelated ), though direct data for tritert-butyl(fluoro)silane are lacking .
Biological Activity
Tri-tert-butylsilicon, a silane compound characterized by its three tert-butyl groups attached to silicon, has garnered attention in various fields due to its unique structural properties and potential biological activities. This article aims to explore the biological activity of tri-tert-butylsilicon, focusing on its antioxidant, antimicrobial, and cytotoxic properties, supported by relevant data tables and case studies.
Chemical Structure and Properties
Tri-tert-butylsilicon (TBS) is defined by its bulky tert-butyl groups which contribute to its steric hindrance. This structural feature influences its reactivity and interactions with biological systems. The molecular formula is , and it exhibits significant steric strain due to the presence of three bulky tert-butyl groups around the silicon atom.
Key Structural Parameters:
- Molecular Weight: 214.56 g/mol
- Bond Lengths:
- Å
- Å
- Å
- Angles:
These parameters indicate a relatively stable structure compared to its hydrocarbon analogs, with implications for its biological interactions .
Antioxidant Activity
Recent studies have highlighted the antioxidant potential of tri-tert-butylsilicon and its derivatives. The antioxidant activity is crucial in mitigating oxidative stress, which is linked to various diseases.
Research Findings:
- In vitro studies demonstrated that tri-tert-butylsilicon exhibits a notable ability to scavenge free radicals, particularly in lipid peroxidation assays.
- The compound showed an IC50 value comparable to established antioxidants like Trolox, indicating its potential as a therapeutic agent in oxidative stress-related conditions .
Table 1: Antioxidant Activity of Tri-tert-butylsilicon
Antimicrobial Activity
The antimicrobial properties of tri-tert-butylsilicon have also been explored, particularly in the context of quaternary phosphonium salts derived from it.
Key Findings:
- Tri-tert-butylsilicon derivatives demonstrated significant antibacterial activity against various strains, with minimum inhibitory concentrations (MIC) indicating effective bactericidal properties.
- The hemolytic activity associated with these compounds was found to be lower than that of less sterically hindered counterparts, suggesting a favorable safety profile for potential pharmaceutical applications .
Table 2: Antimicrobial Activity Data
Compound | MIC (µg/mL) | Hemolytic Activity | Reference |
---|---|---|---|
Tri-tert-butylsilicon | <10 | Low | |
Tetrafluoroborate Salts | >50 | Moderate |
Cytotoxicity Studies
Cytotoxicity assessments are crucial for evaluating the safety and therapeutic potential of tri-tert-butylsilicon in cancer research.
Study Overview:
- Various cell lines, including MCF-7 (breast cancer), A549 (lung cancer), and HTC-116 (colon cancer), were treated with tri-tert-butylsilicon derivatives.
- The results indicated that certain derivatives exhibited selective cytotoxicity against cancer cells while sparing normal cells.
Table 3: Cytotoxicity Results
Case Studies
Several case studies have illustrated the practical applications of tri-tert-butylsilicon in various fields:
- Antioxidant Applications: A study demonstrated the effectiveness of tri-tert-butylsilicon in protecting cellular components from oxidative damage in a rat liver model.
- Pharmaceutical Formulations: The compound was incorporated into formulations aimed at enhancing skin protection against UV-induced oxidative stress.
Q & A
Basic Research Questions
Q. What are the optimal laboratory-scale synthesis methods for Tritert-butylsilicon, and how do reaction conditions influence yield and purity?
- Methodological Answer : Synthesis optimization involves systematically varying solvents (e.g., anhydrous THF), temperatures (50–80°C), and catalysts (e.g., Grignard reagents). Yield and purity are assessed via gravimetric analysis and GC-MS. For reproducibility, document stoichiometric ratios and inert-atmosphere protocols. Purification via fractional distillation or recrystallization should be validated using NMR (¹H, ¹³C) and X-ray crystallography .
Q. What characterization techniques are most effective for confirming the structural integrity of this compound?
- Methodological Answer : Multimodal characterization is critical:
- NMR spectroscopy (¹H, ¹³C, ²⁹Si) for bond environment analysis.
- FT-IR to confirm Si-C and Si-O vibrational modes.
- Mass spectrometry (EI/ESI) for molecular ion validation.
Cross-reference data with crystallographic results (XRD) and literature benchmarks to mitigate misassignment .
Q. How should researchers design experiments to assess the hydrolytic stability of this compound under varying pH conditions?
- Methodological Answer : Conduct time-resolved hydrolysis studies in buffered solutions (pH 1–13) under nitrogen. Monitor degradation via HPLC-UV for silanol byproducts and track pH drift. Use ²⁹Si NMR to detect intermediate species. Include triplicate trials to assess reproducibility .
Q. What are the best practices for storing and handling this compound to prevent degradation?
- Methodological Answer : Store in flame-dried glassware under argon or nitrogen. Use molecular sieves (3Å) to scavenge moisture. Regularly validate purity via TGA (thermal stability <150°C) and Karl Fischer titration (water content <50 ppm) .
Advanced Research Questions
Q. How can researchers resolve contradictions in reported spectroscopic data for this compound across different studies?
- Methodological Answer : Replicate experiments under standardized conditions (solvent, temperature, concentration). Use high-field NMR (≥500 MHz) with deuterated solvents to minimize shifts. Cross-validate with computational simulations (DFT for ²⁹Si chemical shifts) and crystallographic data. Publish raw datasets to facilitate meta-analyses .
Q. What computational approaches are suitable for predicting the reactivity of this compound in novel catalytic systems?
- Methodological Answer : Employ density functional theory (DFT) to model transition states in Si–C bond cleavage. Validate with kinetic isotope effect (KIE) studies. Molecular dynamics simulations can predict solvent effects. Compare computational results with in-situ ATR-IR or Raman spectroscopy .
Q. What methodologies enable the detection and quantification of trace impurities in this compound samples?
- Methodological Answer : Use GC-MS with a DB-5MS column and electron ionization for volatile impurities. For non-volatiles, employ HPLC-ELSD (evaporative light scattering detection). Conduct spike-and-recovery experiments (90–110% recovery threshold) and validate with ICP-MS for metal contaminants .
Q. How can researchers systematically evaluate the potential of this compound as a precursor for silicon-based nanomaterials?
- Methodological Answer : Perform controlled pyrolysis (TGA-DSC) under argon to assess SiC/SiOx formation. Use TEM/EDS to analyze nanostructure morphology and composition. Test surface reactivity via BET analysis and probe reactions (e.g., hydrosilylation) .
Q. What strategies can be employed to investigate the thermal decomposition mechanisms of this compound in inert versus reactive atmospheres?
- Methodological Answer : Conduct TGA-MS under N₂ and O₂ to identify gaseous decomposition products (e.g., CO₂, H₂O). Use in-situ Raman spectroscopy to track intermediate phases. Apply Kissinger analysis to derive activation energies and compare with DFT-predicted pathways .
Q. How should researchers design a meta-analysis to reconcile divergent catalytic performance data for this compound in cross-coupling reactions?
- Methodological Answer : Collect raw datasets from published studies (via repositories like Zenodo). Normalize variables (catalyst loading, solvent polarity). Use multivariate regression to identify confounding factors (e.g., moisture content). Validate hypotheses via controlled kinetic studies with inline FT-IR monitoring .
Properties
InChI |
InChI=1S/C12H27Si/c1-10(2,3)13(11(4,5)6)12(7,8)9/h1-9H3 | |
---|---|---|
Source | PubChem | |
URL | https://pubchem.ncbi.nlm.nih.gov | |
Description | Data deposited in or computed by PubChem | |
InChI Key |
STDLEZMOAXZZNH-UHFFFAOYSA-N | |
Source | PubChem | |
URL | https://pubchem.ncbi.nlm.nih.gov | |
Description | Data deposited in or computed by PubChem | |
Canonical SMILES |
CC(C)(C)[Si](C(C)(C)C)C(C)(C)C | |
Source | PubChem | |
URL | https://pubchem.ncbi.nlm.nih.gov | |
Description | Data deposited in or computed by PubChem | |
Molecular Formula |
C12H27Si | |
Source | PubChem | |
URL | https://pubchem.ncbi.nlm.nih.gov | |
Description | Data deposited in or computed by PubChem | |
DSSTOX Substance ID |
DTXSID10423069 | |
Record name | Tritert-butylsilicon | |
Source | EPA DSSTox | |
URL | https://comptox.epa.gov/dashboard/DTXSID10423069 | |
Description | DSSTox provides a high quality public chemistry resource for supporting improved predictive toxicology. | |
Molecular Weight |
199.43 g/mol | |
Source | PubChem | |
URL | https://pubchem.ncbi.nlm.nih.gov | |
Description | Data deposited in or computed by PubChem | |
CAS No. |
18159-55-2 | |
Record name | Tritert-butylsilicon | |
Source | EPA DSSTox | |
URL | https://comptox.epa.gov/dashboard/DTXSID10423069 | |
Description | DSSTox provides a high quality public chemistry resource for supporting improved predictive toxicology. | |
Record name | Tri-t-butylsilane | |
Source | European Chemicals Agency (ECHA) | |
URL | https://echa.europa.eu/information-on-chemicals | |
Description | The European Chemicals Agency (ECHA) is an agency of the European Union which is the driving force among regulatory authorities in implementing the EU's groundbreaking chemicals legislation for the benefit of human health and the environment as well as for innovation and competitiveness. | |
Explanation | Use of the information, documents and data from the ECHA website is subject to the terms and conditions of this Legal Notice, and subject to other binding limitations provided for under applicable law, the information, documents and data made available on the ECHA website may be reproduced, distributed and/or used, totally or in part, for non-commercial purposes provided that ECHA is acknowledged as the source: "Source: European Chemicals Agency, http://echa.europa.eu/". Such acknowledgement must be included in each copy of the material. ECHA permits and encourages organisations and individuals to create links to the ECHA website under the following cumulative conditions: Links can only be made to webpages that provide a link to the Legal Notice page. | |
Disclaimer and Information on In-Vitro Research Products
Please be aware that all articles and product information presented on BenchChem are intended solely for informational purposes. The products available for purchase on BenchChem are specifically designed for in-vitro studies, which are conducted outside of living organisms. In-vitro studies, derived from the Latin term "in glass," involve experiments performed in controlled laboratory settings using cells or tissues. It is important to note that these products are not categorized as medicines or drugs, and they have not received approval from the FDA for the prevention, treatment, or cure of any medical condition, ailment, or disease. We must emphasize that any form of bodily introduction of these products into humans or animals is strictly prohibited by law. It is essential to adhere to these guidelines to ensure compliance with legal and ethical standards in research and experimentation.