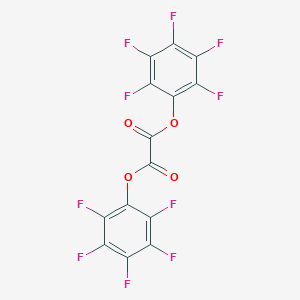
Bis(pentafluorophenyl) oxalate
Overview
Description
Bis(pentafluorophenyl) oxalate is a chemical compound with the molecular formula C₁₄F₁₀O₄ and a molecular weight of 422.13 g/mol . It is commonly used as a chemiluminescence reagent, which means it emits light as a result of a chemical reaction . This compound is particularly known for its application in high-performance liquid chromatography (HPLC) and capillary electrophoresis (CE) due to its sensitivity in detecting fluorescent compounds .
Mechanism of Action
Target of Action
Bis(pentafluorophenyl) Oxalate, also known as PFPO , is primarily used as a chemiluminescence reagent . Its primary targets are fluorescent compounds , which it interacts with to produce a chemiluminescent reaction .
Mode of Action
The compound works by reacting with these fluorescent compounds in the presence of hydrogen peroxide . This reaction results in the emission of light, a process known as chemiluminescence . The emitted light can then be detected and measured, providing valuable information about the target compounds.
Biochemical Pathways
energy transfer processes . In these processes, the energy released from the reaction between this compound and a target compound is transferred to a fluorophore, causing it to emit light .
Result of Action
The primary result of this compound’s action is the production of light through chemiluminescence . This light can be measured and used to quantify the presence of target compounds, making this compound a valuable tool in various research and diagnostic applications.
Action Environment
The efficacy and stability of this compound can be influenced by various environmental factors. For instance, the chemiluminescent reaction it catalyzes requires the presence of hydrogen peroxide . Additionally, factors such as temperature, pH, and the presence of other chemicals can potentially impact the efficiency of this reaction.
Preparation Methods
Synthetic Routes and Reaction Conditions
Bis(pentafluorophenyl) oxalate can be synthesized through the reaction of oxalyl chloride with pentafluorophenol in the presence of a base such as pyridine . The reaction typically occurs under anhydrous conditions to prevent hydrolysis of the reactants and products. The general reaction scheme is as follows:
C2O2Cl2+2C6F5OH→C2O4(C6F5)2+2HCl
Industrial Production Methods
In industrial settings, the production of bis(pentafluorophenyl)oxalate may involve continuous flow processes to enhance efficiency and yield. The use of automated systems ensures precise control over reaction conditions such as temperature, pressure, and reactant concentrations .
Chemical Reactions Analysis
Types of Reactions
Bis(pentafluorophenyl) oxalate primarily undergoes chemiluminescent reactions. When it reacts with hydrogen peroxide in the presence of a fluorescent dye such as 9,10-diphenylanthracene, it produces light . This reaction is highly sensitive and is used in various analytical applications.
Common Reagents and Conditions
Hydrogen Peroxide (H₂O₂): Acts as an oxidizing agent.
Fluorescent Dyes: Such as 9,10-diphenylanthracene, which emit light upon excitation.
Solvents: Organic solvents like acetonitrile or dichloromethane are commonly used.
Major Products
The major product of the chemiluminescent reaction involving bis(pentafluorophenyl)oxalate is light emission at a wavelength of approximately 430 nm .
Scientific Research Applications
Bis(pentafluorophenyl) oxalate has a wide range of applications in scientific research:
Chemistry: Used as a chemiluminescent reagent in HPLC and CE for the sensitive detection of fluorescent compounds.
Biology: Employed in assays to detect the presence of specific biomolecules through chemiluminescence.
Medicine: Utilized in diagnostic tests that rely on chemiluminescent signals to identify disease markers.
Industry: Applied in quality control processes to detect trace amounts of contaminants in various products.
Comparison with Similar Compounds
Bis(pentafluorophenyl) oxalate is unique due to its high sensitivity and efficiency in chemiluminescent reactions. Similar compounds include:
Bis(2,4,6-trichlorophenyl)oxalate: Another chemiluminescent reagent but less sensitive compared to bis(pentafluorophenyl)oxalate.
Bis(4-nitrophenyl)oxalate: Used in similar applications but has different emission characteristics.
These comparisons highlight the superior performance of bis(pentafluorophenyl)oxalate in terms of sensitivity and efficiency in chemiluminescent applications.
Properties
IUPAC Name |
bis(2,3,4,5,6-pentafluorophenyl) oxalate | |
---|---|---|
Source | PubChem | |
URL | https://pubchem.ncbi.nlm.nih.gov | |
Description | Data deposited in or computed by PubChem | |
InChI |
InChI=1S/C14F10O4/c15-1-3(17)7(21)11(8(22)4(1)18)27-13(25)14(26)28-12-9(23)5(19)2(16)6(20)10(12)24 | |
Source | PubChem | |
URL | https://pubchem.ncbi.nlm.nih.gov | |
Description | Data deposited in or computed by PubChem | |
InChI Key |
YMTUYHWOWDFXOX-UHFFFAOYSA-N | |
Source | PubChem | |
URL | https://pubchem.ncbi.nlm.nih.gov | |
Description | Data deposited in or computed by PubChem | |
Canonical SMILES |
C1(=C(C(=C(C(=C1F)F)F)F)F)OC(=O)C(=O)OC2=C(C(=C(C(=C2F)F)F)F)F | |
Source | PubChem | |
URL | https://pubchem.ncbi.nlm.nih.gov | |
Description | Data deposited in or computed by PubChem | |
Molecular Formula |
C14F10O4 | |
Source | PubChem | |
URL | https://pubchem.ncbi.nlm.nih.gov | |
Description | Data deposited in or computed by PubChem | |
DSSTOX Substance ID |
DTXSID60371102 | |
Record name | Bis(pentafluorophenyl) oxalate | |
Source | EPA DSSTox | |
URL | https://comptox.epa.gov/dashboard/DTXSID60371102 | |
Description | DSSTox provides a high quality public chemistry resource for supporting improved predictive toxicology. | |
Molecular Weight |
422.13 g/mol | |
Source | PubChem | |
URL | https://pubchem.ncbi.nlm.nih.gov | |
Description | Data deposited in or computed by PubChem | |
CAS No. |
16536-48-4 | |
Record name | Bis(pentafluorophenyl) oxalate | |
Source | EPA DSSTox | |
URL | https://comptox.epa.gov/dashboard/DTXSID60371102 | |
Description | DSSTox provides a high quality public chemistry resource for supporting improved predictive toxicology. | |
Retrosynthesis Analysis
AI-Powered Synthesis Planning: Our tool employs the Template_relevance Pistachio, Template_relevance Bkms_metabolic, Template_relevance Pistachio_ringbreaker, Template_relevance Reaxys, Template_relevance Reaxys_biocatalysis model, leveraging a vast database of chemical reactions to predict feasible synthetic routes.
One-Step Synthesis Focus: Specifically designed for one-step synthesis, it provides concise and direct routes for your target compounds, streamlining the synthesis process.
Accurate Predictions: Utilizing the extensive PISTACHIO, BKMS_METABOLIC, PISTACHIO_RINGBREAKER, REAXYS, REAXYS_BIOCATALYSIS database, our tool offers high-accuracy predictions, reflecting the latest in chemical research and data.
Strategy Settings
Precursor scoring | Relevance Heuristic |
---|---|
Min. plausibility | 0.01 |
Model | Template_relevance |
Template Set | Pistachio/Bkms_metabolic/Pistachio_ringbreaker/Reaxys/Reaxys_biocatalysis |
Top-N result to add to graph | 6 |
Feasible Synthetic Routes
Q1: What role does Bis(pentafluorophenyl)oxalate play in the detection of estradiol in plasma?
A: Bis(pentafluorophenyl)oxalate (PFPO) acts as a chemiluminescent reagent in the detection method described in the research paper []. It is not a target of estradiol or involved in its biological pathways. Instead, PFPO participates in a chemical reaction that generates light when it reacts with a derivative of estradiol (dansylated-estradiol) in the presence of hydrogen peroxide. This light emission is then measured to determine the concentration of estradiol in the sample.
Disclaimer and Information on In-Vitro Research Products
Please be aware that all articles and product information presented on BenchChem are intended solely for informational purposes. The products available for purchase on BenchChem are specifically designed for in-vitro studies, which are conducted outside of living organisms. In-vitro studies, derived from the Latin term "in glass," involve experiments performed in controlled laboratory settings using cells or tissues. It is important to note that these products are not categorized as medicines or drugs, and they have not received approval from the FDA for the prevention, treatment, or cure of any medical condition, ailment, or disease. We must emphasize that any form of bodily introduction of these products into humans or animals is strictly prohibited by law. It is essential to adhere to these guidelines to ensure compliance with legal and ethical standards in research and experimentation.