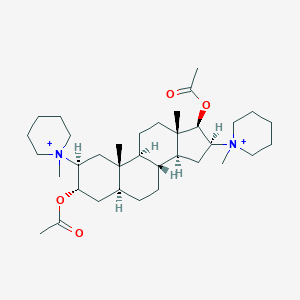
Pancuronium
Overview
Description
Pancuronium bromide is a non-depolarizing aminosteroid neuromuscular blocking agent (NMBA) widely used in clinical anesthesia to induce skeletal muscle relaxation during surgery and mechanical ventilation . It functions primarily as a competitive antagonist at nicotinic acetylcholine receptors (nAChRs) at the neuromuscular junction, preventing acetylcholine-induced depolarization. However, recent studies in zebrafish models suggest this compound may also act via allosteric inhibition, independent of the classical ligand-binding site . This compound exhibits a prolonged duration of action compared to other NMBAs, with a slower spontaneous recovery profile, necessitating pharmacologic reversal (e.g., neostigmine) in clinical settings . Notably, it has been controversially employed in lethal injection protocols due to its paralytic effects .
Preparation Methods
Synthetic Routes and Reaction Conditions: Pancuronium is synthesized through a series of chemical reactions involving steroidal precursorsThe reaction conditions often require precise control of temperature, pH, and the use of specific catalysts to ensure the correct formation of the desired product .
Industrial Production Methods: In industrial settings, this compound is produced using large-scale chemical reactors where the reaction conditions are meticulously controlled to maximize yield and purity. The process involves multiple purification steps, including crystallization and chromatography, to remove impurities and obtain pharmaceutical-grade this compound .
Chemical Reactions Analysis
Hydrolysis Reactions and Degradation Pathways
Pancuronium bromide is susceptible to hydrolysis under acidic, alkaline, and thermal conditions, leading to the formation of desacetyl metabolites. These reactions are critical for understanding its stability and degradation profile:
Key Degradation Products
Compound Name | CAS Number | Conditions Leading to Formation |
---|---|---|
3-desacetylthis compound | 43021–44–9 | Acidic (HCl), alkaline (NaOH), heat |
17-desacetylthis compound | 27115–86–2 | Acidic, alkaline, heat |
3,17-didesacetylthis compound | 43021–46–1 | Sequential hydrolysis of 3-/17-dPC |
Mechanism :
-
Acidic/Basic Hydrolysis : Cleavage of acetyl groups at the 3- or 17-position of the androstane skeleton (Figure 1 in ).
-
Thermal Degradation : Accelerates hydrolysis even in neutral buffers, forming 3-desacetylthis compound (3-dPC) and 17-desacetylthis compound (17-dPC) as intermediates.
Forced Degradation Studies
Condition | Degradation Products Identified |
---|---|
0.1–1 M HCl | 3-dPC, 17-dPC, 3,17-ddPC |
0.01–0.1 M NaOH | 3-dPC, 17-dPC, 3,17-ddPC |
80°C for 96 hrs | 3-dPC, 17-dPC, 3,17-ddPC |
3% H₂O₂ | No notable degradation |
Data from forced degradation experiments reveal that oxidative stress (H₂O₂) and UV exposure do not produce significant degradation products .
Stability in Buffered Solutions
This compound bromide exhibits pH-dependent stability in saline-buffered solutions. A novel HPLC-MS method quantified its degradation kinetics:
Stability-Indicating Parameters
Parameter | Value (Validation) |
---|---|
Linearity (R²) | 0.985 (0.5–2.5 µg/mL range) |
Repeatability (RSD) | 4.68% |
Reproducibility (RSD) | 4.52% |
Trueness | -1.01% |
The study confirmed that this compound bromide remains stable for 24 hours at room temperature but degrades progressively under extreme pH or heat .
Metabolic Pathways
In vivo, this compound undergoes hepatic and renal metabolism, producing pharmacologically active and inactive metabolites:
Major Metabolites
-
3-Hydroxy metabolite : 25% of injected dose recovered; half the potency of this compound .
-
3,17-Dihydroxy metabolite : Trace amounts; negligible activity .
Excretion Profile
Route | Percentage Excreted | Components |
---|---|---|
Urine | 40% | Unchanged this compound + metabolites |
Bile | 11% | Unchanged this compound + metabolites |
Patients with hepatic cirrhosis or biliary obstruction show altered pharmacokinetics, including doubled elimination half-life and reduced plasma clearance .
Analytical Methods for Degradation Analysis
A validated HPLC-MS method identified degradation products using specific ion fragments:
MS Parameters for Detection
Compound | Retention Time (min) | Ion (m/z) | Fragmentor Voltage (V) |
---|---|---|---|
This compound | 1.40 | 286.2 | 110 |
3-dPC | 1.35 | 265.2 | 110 |
17-dPC | 1.45 | 265.2 | 110 |
3,17-ddPC | 1.15 | 244.2 | 110 |
This method enabled precise quantification of this compound and its impurities, meeting European Pharmacopoeia criteria .
Comparative Reactivity with Analogues
This compound’s hydrolysis profile contrasts with newer neuromuscular blockers:
Scientific Research Applications
Clinical Applications
-
Anesthesia : Pancuronium is primarily used as an adjunct to general anesthesia. Its applications include:
- Facilitating Intubation : It provides effective muscle relaxation that aids in endotracheal intubation, ensuring airway management during surgery .
- Skeletal Muscle Relaxation : this compound allows for optimal surgical conditions by relaxing skeletal muscles, which is essential for various surgical procedures .
- Mechanical Ventilation : In critically ill patients, this compound can improve thoracic compliance and assist in mechanical ventilation by relaxing chest wall and diaphragmatic muscles .
- Therapeutic Hypothermia : this compound has been utilized in protocols for therapeutic hypothermia following cardiac arrest to prevent shivering, which can complicate temperature management during resuscitation efforts .
- Acute Respiratory Distress Syndrome (ARDS) : While not extensively studied, there is potential for this compound to decrease inflammatory responses associated with ARDS when used as part of a mechanical ventilation strategy .
Pharmacokinetics
This compound's pharmacokinetic profile includes:
- Elimination : Primarily renally excreted (80%), with minor contributions from hepatic metabolism (10%) and biliary excretion (10%). This renal clearance necessitates caution in patients with renal impairment .
- Volume of Distribution : The volume of distribution is nearly equivalent to circulating plasma volume due to its poor lipid solubility, preventing it from crossing the blood-brain barrier .
Veterinary Applications
This compound is also employed in veterinary medicine, particularly for the immobilization of large animals such as crocodiles. Its use allows for safe handling during medical procedures or research activities. The following table summarizes a study on the use of this compound for immobilizing Nile crocodiles:
Sex | Body Weight (kg) | Total Length (cm) | Cloacal Temperature (°C) | This compound Bromide (mg/kg) | Induction Time (min) | Recovery Time |
---|---|---|---|---|---|---|
M | 54 | 220 | 19.0 | 0.019 | 20 | 50 min |
F | 130 | 275 | 20.5 | 0.015 | 20 | 50 min |
The above data indicates that this compound effectively immobilizes adult Nile crocodiles while allowing for predictable recovery times when reversed with neostigmine .
Case Studies and Research Findings
Numerous studies have documented the efficacy and safety of this compound in clinical settings:
- A clinical study demonstrated that this compound could serve as the sole muscle relaxant during major surgeries with predictable recovery profiles .
- Another investigation highlighted its role in improving outcomes for patients undergoing mechanical ventilation due to respiratory failure, although specific studies on this compound's effects compared to other neuromuscular blockers remain limited .
Mechanism of Action
Pancuronium exerts its effects by competitively inhibiting the nicotinic acetylcholine receptors at the neuromuscular junction. This inhibition prevents acetylcholine from binding to its receptors, thereby reducing the response of the end plate to acetylcholine and leading to muscle relaxation . This compound has slight vagolytic activity, causing an increase in heart rate, but no ganglioplegic activity .
Comparison with Similar Compounds
Comparison with Similar Compounds
Pancuronium is part of the aminosteroid class of NMBAs, which includes vecuronium, rocuronium, and pipecuronium. Its pharmacodynamic and pharmacokinetic properties are distinct from benzylisoquinolinium agents (e.g., cisatracurium, atracurium). Below is a detailed comparison:
Vecuronium
- Structural and Transcriptomic Profile : Despite structural identity (Tanimoto coefficient = 1) with this compound, vecuronium induces divergent transcriptomic responses in human cells, regulating oxidation reduction-related genes versus this compound’s effects on immune and mitochondrial pathways .
- Receptor Binding : Both drugs competitively inhibit nAChRs but exhibit partial competition when co-administered with (+)-tubocurarine. This compound shows a 9–29-fold preference for the αδ-interface of nAChRs, while vecuronium shares similar site selectivity .
- Clinical Use : Vecuronium has a shorter duration and faster recovery than this compound, reducing residual neuromuscular blockade risks .
Rocuronium
- Receptor Specificity : Unlike this compound, rocuronium shows minimal preference for nAChR subunit interfaces, with mutations in both ε- and δ-subunits affecting its potency .
- Clinical Outcomes : In "fast-track" cardiac surgery, rocuronium’s duration is more predictable than this compound, which is associated with prolonged paralysis and residual block .
Cisatracurium
- Pharmacodynamics: Cisatracurium, a benzylisoquinolinium compound, has a 4-fold lower potency than this compound but a faster spontaneous recovery (T1% to 5%: ~20 min vs. ~30 min for this compound) .
- Site Selectivity : Unlike this compound’s αδ-interface preference, cisatracurium favors the α-ε interface by a 3–7-fold margin .
Pipecuronium
- Potency : Pipecuronium is 20% more potent than this compound in clinical studies .
- Cardiovascular Effects : Unlike this compound’s tachycardia, pipecuronium causes mild bradycardia and exhibits stable hemodynamics in cardiac patients .
Doxacurium
- ICU Applications : Doxacurium, administered via continuous infusion, offers predictable neuromuscular blockade with fewer hemodynamic fluctuations compared to this compound .
Pharmacokinetic and Pharmacodynamic Data
Mechanistic and Clinical Considerations
- Allosteric Inhibition : this compound’s action in zebrafish nAChRs involves allosteric modulation, contrasting with traditional competitive antagonism in mammals .
- Sodium Channel Effects : this compound immobilizes gating currents in squid axons, akin to N-methyl-strychnine (NMS) and QX-314, suggesting a shared mechanism of intracellular sodium channel blockade .
- Reversal Agents : Neostigmine effectively reverses this compound, cisatracurium, and vecuronium, while l-cysteine accelerates recovery for newer agents like CW002 .
Clinical Implications
- Cardiac Surgery : Pipecuronium and rocuronium are preferred over this compound for hemodynamic stability and predictable recovery .
- ICU Use : this compound’s prolonged action increases residual blockade risks, making cisatracurium or vecuronium safer for long-term paralysis .
- Controversies : this compound’s role in lethal injection protocols underscores ethical concerns due to its paralytic masking of consciousness during potassium chloride-induced cardiac arrest .
Biological Activity
Pancuronium bromide is a long-acting, non-depolarizing neuromuscular blocking agent (NMBA) widely used in anesthesia to facilitate intubation and provide muscle relaxation during surgical procedures. Its mechanism of action, pharmacological effects, and clinical implications are critical for understanding its biological activity.
This compound acts primarily as a competitive antagonist at the neuromuscular junction by binding to nicotinic acetylcholine receptors (nAChRs) on the motor end plate, thereby preventing acetylcholine from eliciting muscle contraction. This blockade results in muscle paralysis without the initial muscle fasciculations seen with depolarizing agents like succinylcholine .
Key Targets
- Neuronal Acetylcholine Receptor Subunit Alpha-2 : this compound binds to this receptor, inhibiting its activity .
- Muscarinic Acetylcholine Receptors (M2 and M3) : Although the exact pharmacological actions of this compound on these receptors are not fully understood, it is known to exert some antagonistic effects .
Pharmacokinetics
This compound exhibits a relatively long duration of action due to its pharmacokinetic profile. It is eliminated primarily through renal pathways, with a half-life ranging from 1.5 to 3 hours in healthy individuals. The onset of action typically occurs within 2-4 minutes after intravenous administration .
Pharmacokinetic Parameter | Value |
---|---|
Half-Life | 1.5 - 3 hours |
Onset Time | 2 - 4 minutes |
Duration of Action | 60 - 90 minutes |
Cardiovascular Effects
This compound has been shown to increase heart rate in a dose-dependent manner, which can be clinically significant, especially in patients with pre-existing cardiovascular conditions. In studies, it was noted that this compound increased heart rate from a baseline of approximately 103 bpm to about 112 bpm at higher doses .
Group | Heart Rate (bpm) | Developed Force |
---|---|---|
Control | 103.6 (±2.9) | 78.2 (±7.2) |
This compound | 111.7 (±3.0)* | 90.4 (±6.0)* |
This compound + Propranolol | 106.4 (±2.5) | 83.2 (±6.4) |
*Significant difference from control (P < 0.05) .
Case Study: Defasciculating Doses
A study investigated the effects of low-dose this compound as a defasciculating agent prior to succinylcholine administration. Results indicated that pre-treatment with this compound significantly prolonged the duration of succinylcholine-induced paralysis, suggesting that its use can enhance the efficacy of succinylcholine without necessitating higher doses .
- Duration of Action :
- Succinylcholine alone: Recovery after approximately 12 minutes.
- Succinylcholine with this compound: Recovery after approximately 15-18 minutes.
This finding emphasizes the importance of dosing strategies in clinical practice to optimize neuromuscular blockade while minimizing side effects.
Safety and Side Effects
This compound is generally well-tolerated; however, it can cause cardiovascular side effects such as tachycardia and hypertension due to its vagolytic properties . Close monitoring is required in patients with cardiovascular comorbidities.
Q & A
Basic Research Questions
Q. What experimental models are used to study pancuronium’s neuromuscular blocking efficacy in conscious vs. anesthetized subjects?
- Methodology: In conscious volunteers, this compound’s effects are measured via thumb adduction response (e.g., using force-displacement transducers) under controlled neuromuscular stimulation. Anesthetized models involve monitoring tidal volume, arterial blood gases, and indirect muscle stimulation (e.g., adductor pollicis). Dose-response curves are constructed using cumulative dosing or single-bolus methods, with statistical analysis (e.g., log-probit or linear regression) to determine ED50/ED95 .
- Key Data: In conscious subjects, 40 µg/kg this compound reduced twitch tension by 11.5±2.8%, recovering to 66.9±6.0% after 60 minutes .
Q. How do pharmacokinetic parameters of this compound vary between patients with normal renal function and those with chronic renal failure?
- Methodology: Plasma concentrations are measured using fluorimetry (accuracy: ±0.02 µg/mL) in two-compartment pharmacokinetic models. Statistical comparisons (e.g., unpaired t-tests) assess clearance rates and central compartment volumes. Urinary excretion is correlated with creatinine clearance via Spearman tests .
- Key Data: Clearance decreases by ~50% in renal failure patients, with central compartment volume increasing significantly (p<0.01) .
Advanced Research Questions
Q. How does hypothermia during cardiopulmonary bypass (CPB) influence this compound’s plasma concentration and neuromuscular blockade dynamics?
- Methodology: this compound requirements are monitored during CPB with hypothermia (24–26°C rectal temperature). Plasma and urinary concentrations are sampled every 15 minutes and analyzed via spectrofluorimetry. Variance analysis and Bonferroni t-tests compare periods (pre-CPB, cooling, rewarming) .
- Key Data: this compound demand remains stable at 10±3% of initial value during hypothermia, but plasma concentrations rise during rewarming (0.20±0.04 µg/mL at 25% recovery) .
Q. What methodological challenges arise when analyzing dose-response curves for this compound, and how do log-probit vs. linear regression approaches compare?
- Methodology: Cumulative dose-response data are plotted on log-probit scales or arithmetic graphs. The Litchfield-Wilcoxon method determines ED50/ED95, validated against linear regression. Incremental dosing efficiency is tested against single-bolus methods .
- Key Data: Log-probit analysis better fits extreme responses (85–99% blockade), with no statistical difference in ED50/ED95 between methods (this compound ED95: ~0.06 mg/kg) .
Q. How do contradictory findings on this compound’s impact on postoperative intubation time in cardiac surgery inform study design?
- Methodology: Conflicting results (e.g., prolonged intubation vs. safe early extubation) require stratified analysis of variables: CPB duration, residual blockade monitoring (train-of-four ratio), and co-administered anesthetics. Retrospective cohort studies with multivariate regression isolate this compound’s role .
- Key Data: Residual blockade persists up to 8 hours post-CPB, necessitating quantitative monitoring (e.g., acceleromyography) .
Q. What statistical approaches resolve contradictions in this compound’s diaphragmatic effects (e.g., force enhancement vs. central apnea)?
- Methodology: Direct measurement of diaphragmatic pressure (Pdi) via esophageal balloons and needle thermometers. Multivariate analysis accounts for confounding factors (e.g., aminophylline co-administration, anesthesia depth) .
- Key Data: this compound increases Pdi in hypoxic conditions but reduces force output in normoxia, highlighting context-dependent effects .
Q. Methodological Considerations for Data Interpretation
Q. How reliable are fluorimetric assays for this compound quantification, and what are their limitations in distinguishing metabolites?
- Methodology: Fluorimetry (sensitivity: 0.02 µg/mL) measures total this compound + metabolites. Cross-validation with thin-layer chromatography (TLC) or HPLC improves specificity, but TLC’s semiquantitative nature limits precision .
- Key Data: Fluorimetry overestimates active drug levels by 20–30% due to 3-hydroxy metabolite interference .
Q. What experimental designs minimize bias when studying this compound’s interaction with inhalation anesthetics (e.g., halothane)?
- Methodology: Controlled alveolar anesthetic concentrations (e.g., via gas chromatography) standardize neuromuscular blockade comparisons. Randomized crossover designs mitigate inter-subject variability .
- Key Data: Halothane potentiates this compound’s blockade by 30–40%, requiring dose adjustments in balanced anesthesia .
Q. Contradictions and Future Directions
- Elimination Pathway Discrepancies : While renal excretion accounts for 37–44% of this compound, biliary excretion (11%) and metabolite dynamics require isotope-tracing studies .
- Muscarinic Receptor Interactions : Structural analogs (e.g., vecuronium) with reduced M2-blocking effects suggest rational redesign opportunities, but this compound’s steroid skeleton remains a template for potency studies .
Properties
CAS No. |
16974-53-1 |
---|---|
Molecular Formula |
C35H60N2O4+2 |
Molecular Weight |
572.9 g/mol |
IUPAC Name |
[(2S,3S,5S,8R,9S,10S,13S,14S,16S,17R)-17-acetyloxy-10,13-dimethyl-2,16-bis(1-methylpiperidin-1-ium-1-yl)-2,3,4,5,6,7,8,9,11,12,14,15,16,17-tetradecahydro-1H-cyclopenta[a]phenanthren-3-yl] acetate |
InChI |
InChI=1S/C35H60N2O4/c1-24(38)40-32-21-26-13-14-27-28(35(26,4)23-31(32)37(6)19-11-8-12-20-37)15-16-34(3)29(27)22-30(33(34)41-25(2)39)36(5)17-9-7-10-18-36/h26-33H,7-23H2,1-6H3/q+2/t26-,27+,28-,29-,30-,31-,32-,33-,34-,35-/m0/s1 |
InChI Key |
GVEAYVLWDAFXET-XGHATYIMSA-N |
SMILES |
CC(=O)OC1CC2CCC3C(C2(CC1[N+]4(CCCCC4)C)C)CCC5(C3CC(C5OC(=O)C)[N+]6(CCCCC6)C)C |
Isomeric SMILES |
CC(=O)O[C@H]1C[C@@H]2CC[C@@H]3[C@@H]([C@]2(C[C@@H]1[N+]4(CCCCC4)C)C)CC[C@]5([C@H]3C[C@@H]([C@@H]5OC(=O)C)[N+]6(CCCCC6)C)C |
Canonical SMILES |
CC(=O)OC1CC2CCC3C(C2(CC1[N+]4(CCCCC4)C)C)CCC5(C3CC(C5OC(=O)C)[N+]6(CCCCC6)C)C |
Color/Form |
Crystals WHITE POWDER |
melting_point |
215 °C |
Key on ui other cas no. |
16974-53-1 15500-66-0 |
physical_description |
Solid |
Pictograms |
Acute Toxic |
shelf_life |
SENSITIVE TO HEAT ... The manufacturer indicates that the drug is stable for 6 mos at room temperature. |
solubility |
1 g sol in 30 parts chloroform, 1 part water (20 °C) SOL IN ALCOHOL Very soluble in water. 3.08e-06 g/L |
Synonyms |
Bromide, Pancuronium Pancuronium Pancuronium Bromide Pancuronium Curamed Pancuronium Organon Pavulon |
Origin of Product |
United States |
Retrosynthesis Analysis
AI-Powered Synthesis Planning: Our tool employs the Template_relevance Pistachio, Template_relevance Bkms_metabolic, Template_relevance Pistachio_ringbreaker, Template_relevance Reaxys, Template_relevance Reaxys_biocatalysis model, leveraging a vast database of chemical reactions to predict feasible synthetic routes.
One-Step Synthesis Focus: Specifically designed for one-step synthesis, it provides concise and direct routes for your target compounds, streamlining the synthesis process.
Accurate Predictions: Utilizing the extensive PISTACHIO, BKMS_METABOLIC, PISTACHIO_RINGBREAKER, REAXYS, REAXYS_BIOCATALYSIS database, our tool offers high-accuracy predictions, reflecting the latest in chemical research and data.
Strategy Settings
Precursor scoring | Relevance Heuristic |
---|---|
Min. plausibility | 0.01 |
Model | Template_relevance |
Template Set | Pistachio/Bkms_metabolic/Pistachio_ringbreaker/Reaxys/Reaxys_biocatalysis |
Top-N result to add to graph | 6 |
Feasible Synthetic Routes
Disclaimer and Information on In-Vitro Research Products
Please be aware that all articles and product information presented on BenchChem are intended solely for informational purposes. The products available for purchase on BenchChem are specifically designed for in-vitro studies, which are conducted outside of living organisms. In-vitro studies, derived from the Latin term "in glass," involve experiments performed in controlled laboratory settings using cells or tissues. It is important to note that these products are not categorized as medicines or drugs, and they have not received approval from the FDA for the prevention, treatment, or cure of any medical condition, ailment, or disease. We must emphasize that any form of bodily introduction of these products into humans or animals is strictly prohibited by law. It is essential to adhere to these guidelines to ensure compliance with legal and ethical standards in research and experimentation.