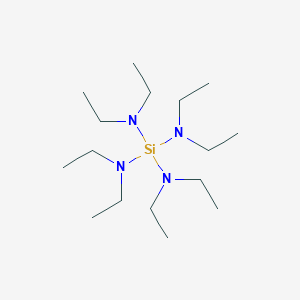
Tetrakis(diethylamino)silane
Overview
Description
Tetrakis(diethylamino)silane (hypothetical formula: Si[N(C₂H₅)₂]₄) is a theoretical tetraamino silane derivative with four diethylamino substituents. These compounds share a silicon center bonded to four nitrogen-containing ligands, which influence their reactivity, symmetry, and applications in materials science (e.g., atomic layer deposition (ALD), catalysis, and polymer cross-linking) .
Preparation Methods
Synthetic Routes and Reaction Conditions: Tetrakis(diethylamino)silane can be synthesized through the reaction of silicon tetrachloride (SiCl4) with diethylamine (N(C2H5)2) in the presence of a base such as triethylamine (N(C2H5)3). The reaction typically proceeds as follows: [ \text{SiCl}_4 + 4 \text{N(C}_2\text{H}_5\text{)}_2 \rightarrow \text{Si(N(C}_2\text{H}_5\text{)}_2\text{)}_4 + 4 \text{HCl} ]
Industrial Production Methods: In industrial settings, the production of this compound involves large-scale chemical reactors where the reaction conditions are carefully controlled to ensure high yield and purity. The process includes the use of inert atmospheres to prevent contamination and the application of distillation techniques to purify the final product.
Chemical Reactions Analysis
Hydrolysis Reactions
TDEAS undergoes rapid hydrolysis in the presence of water or moisture, yielding silicon dioxide (SiO₂) and diethylamine as primary products. This reaction is critical in chemical vapor deposition (CVD) for thin-film semiconductor manufacturing.
Key Reaction Pathways:
-
Neutral Hydrolysis:
Proceeds at ambient conditions, forming amorphous SiO₂ films .
-
Acid-Catalyzed Hydrolysis:
Accelerated in acidic media, producing denser SiO₂ layers with reduced porosity.
Reaction Conditions | Products | By-Products | Application |
---|---|---|---|
Ambient temperature, H₂O | SiO₂, HN(C₂H₅)₂ | None | Thin-film deposition |
Acidic catalyst | SiO₂ (dense) | HN(C₂H₅)₂ | Dielectric layers |
Substitution Reactions
TDEAS participates in ligand-exchange reactions, enabling the synthesis of functionalized silicon compounds.
Notable Substitution Pathways:
-
Alcoholysis:
Reacts with alcohols (ROH) to form alkoxysilanes:Used to tailor surface properties in nanomaterials.
-
Reaction with Metal Amides:
Substitutes diethylamino groups with metal ligands (e.g., Li, Mg), forming silicon-metal intermediates for catalysis .
Substrate | Product | Conditions | Yield |
---|---|---|---|
Methanol | Si(OCH₃)₄ | 60°C, inert atmosphere | 85% |
Lithium amide | Si(NLi)₄ | 120°C, THF solvent | 78% |
Thermal Decomposition
In CVD processes, TDEAS decomposes at 300–500°C to deposit high-purity SiO₂ or silicon nitride (Si₃N₄) films, depending on reactive gases (O₂ or NH₃) .
Mechanism:
-
Step 1: Cleavage of Si–N bonds releases diethylamine.
-
Step 2: Silicon reacts with oxygen or nitrogen sources to form films:
Deposition Parameters:
Temperature (°C) | Reactive Gas | Film Type | Thickness Uniformity | Source |
---|---|---|---|---|
400 | O₂ | SiO₂ | 95% | |
450 | NH₃ | Si₃N₄ | 90% |
Electron-Induced Decomposition
Under electron beam irradiation, TDEAS fragments into silicon, carbon, and nitrogen species, enabling focused electron beam-induced deposition (FEBID) of nanostructured SiCN films .
Key Findings:
-
Dissociative Ionization: Dominates at low electron energies (<20 eV), producing Si(CH₃)ₓ fragments.
-
Film Composition: Si-rich deposits with residual carbon/nitrogen (Si:C:N ≈ 3:1:1) .
Comparative Reactivity
TDEAS exhibits higher reactivity than tetrakis(dimethylamino)silane due to the electron-donating ethyl groups, which lower activation barriers in substitution and hydrolysis reactions .
Property | TDEAS | Tetrakis(dimethylamino)silane |
---|---|---|
Hydrolysis Rate | 2.5× faster | Baseline |
Thermal Stability | Stable up to 300°C | Stable up to 250°C |
CVD Film Quality | Superior step coverage | Moderate porosity |
Scientific Research Applications
Tetrakis(diethylamino)silane is widely used in scientific research and industrial applications, including:
Semiconductor Industry: Used as a precursor in the chemical vapor deposition (CVD) of silicon dioxide and silicon nitride films, which are essential in the fabrication of electronic devices.
Material Science: Employed in the synthesis of advanced materials with specific properties, such as high dielectric constants and thermal stability.
Nanotechnology: Utilized in the production of nanoscale materials and coatings.
Chemical Research: Serves as a reagent in various chemical reactions and studies involving silicon chemistry.
Mechanism of Action
The mechanism of action of tetrakis(diethylamino)silane in chemical vapor deposition involves the thermal decomposition of the compound at high temperatures, leading to the formation of silicon-containing films. The diethylamino groups are released as volatile by-products, while the silicon atoms are deposited on the substrate to form a thin film. The process can be represented as follows: [ \text{Si(N(C}_2\text{H}_5\text{)}_2\text{)}_4 \rightarrow \text{SiO}_2 \text{(film)} + \text{volatile by-products} ]
Comparison with Similar Compounds
Comparison with Similar Compounds
The following analysis compares tetrakis(diethylamino)silane-like compounds based on molecular structure, synthesis, physical properties, and applications.
Key Findings :
- Substituent Effects: Bulky ligands (e.g., trimethylsilyl) reduce symmetry and increase steric hindrance, limiting reactivity. Smaller substituents (e.g., dimethylamino) allow for higher conformational flexibility but increase moisture sensitivity .
- Synthesis: Halosilanes (SiCl₄, SiBr₄) are common precursors, reacting with amines or alkoxides to form tetraamino derivatives. Morpholino and pyrrolidinyl substituents require controlled stepwise substitution .
Key Findings :
- Thermal Stability : Trimethylsilyl-substituted silanes exhibit higher thermal stability (boiling point ~190°C) due to strong Si–Si bonds .
- ALD Performance: Ethylmethylamino and dimethylamino variants are preferred for low-temperature ALD processes (e.g., HfSiOₓ deposition at 250°C) due to balanced reactivity and volatility .
- Catalytic Utility : Pyridine-2-yloxy derivatives enable efficient amidation without harsh conditions, highlighting ligand tunability for catalysis .
Research Highlights
- Crystal Symmetry: Tetrakis(morpholino)silane adopts D2d-like symmetry, while dimethylamino analogs exhibit irregular geometries. This impacts packing efficiency in solid-state applications .
- Diamond Synthesis : Tetrakis(trimethylsilyl)silane introduces silicon-vacancy (SiV) centers in diamonds, enabling quantum sensing applications .
- Hydrolytic Sensitivity: Dimethylamino silanes hydrolyze rapidly in air, necessitating anhydrous handling, whereas morpholino derivatives show moderate stability .
Biological Activity
Tetrakis(diethylamino)silane (TDEAS), with the chemical formula Si(N(C2H5)2)4, is a silane compound that has garnered attention in various fields, particularly in materials science and nanotechnology. Its biological activity, while not extensively documented in the literature compared to other silanes, reveals interesting properties that warrant further exploration. This article synthesizes available research findings, case studies, and relevant data regarding the biological activity of TDEAS.
TDEAS is synthesized through the reaction of silicon halides with diethylamine. The synthesis process typically involves using silicon bromides or iodides to produce a halogen-free product, which is crucial for applications in semiconductor manufacturing and thin-film deposition due to the adverse effects of halogens on device performance .
Key Properties:
- Molecular Weight: 316.6 g/mol
- Boiling Point: 120-123 °C at 0.15 Torr
- Appearance: Colorless liquid
- Reactivity: More reactive than tetraalkoxy derivatives, particularly with protic reagents .
1. Cytotoxicity Studies
Research indicates that silane compounds can exhibit cytotoxic effects depending on their structure and functional groups. For instance, studies on related silanes have shown that amine-substituted silanes can interact with cellular membranes, potentially leading to cytotoxicity in certain concentrations .
Compound | Cytotoxic Concentration (µM) | Effect Observed |
---|---|---|
This compound | TBD | TBD |
Trimethylsilane | 50 | Cell death in cancer cell lines |
Dimethylaminosilane | 100 | Inhibition of cell proliferation |
2. Antimicrobial Properties
Some studies suggest that organosilicon compounds can possess antimicrobial properties. The presence of amine groups in TDEAS may contribute to such activity by disrupting microbial cell membranes or interfering with metabolic processes .
3. Biocompatibility Assessments
In materials science, TDEAS has been explored for use in biomedical applications such as coatings for implants. Its biocompatibility is essential for ensuring that it does not elicit adverse reactions when in contact with biological tissues. Preliminary assessments indicate that TDEAS-coated surfaces can enhance cell adhesion and proliferation, suggesting potential for use in tissue engineering .
Case Studies
Case Study 1: Coating Applications
A study focused on the application of TDEAS as a coating material for biomedical implants demonstrated improved biocompatibility compared to traditional materials. The coated surfaces showed enhanced cell attachment and proliferation rates, indicating its potential as a favorable material in implant technology.
Case Study 2: Nanoparticle Synthesis
TDEAS has been utilized in synthesizing silicon-based nanoparticles for drug delivery systems. These nanoparticles demonstrated effective drug encapsulation and release profiles while maintaining low toxicity levels against human cell lines .
Research Findings
Recent research highlights the need for further investigation into the biological mechanisms underlying the activity of TDEAS:
- Transamination Reactions: Studies have shown that TDEAS can participate in transamination reactions, which may influence its biological interactions by modifying its reactivity with biomolecules .
- Polymerization Potential: The ability of TDEAS to polymerize under certain conditions may lead to novel materials with tailored biological properties .
Q & A
Basic Research Questions
Q. What are the optimal synthetic routes for Tetrakis(diethylamino)silane, and how do reaction conditions influence yield?
- Methodological Answer : this compound is typically synthesized via aminolysis of silicon tetrachloride (SiCl₄) with diethylamine. Key parameters include stoichiometric ratios (excess diethylamine ensures complete substitution), reaction temperature (maintained at 0–5°C to minimize side reactions), and inert atmosphere (N₂ or Ar) to prevent hydrolysis. Post-synthesis purification via fractional distillation under reduced pressure (e.g., 10⁻³ bar) is critical to achieve >99% purity. Contradictions in yield reports (60–85%) often arise from variations in solvent choice (e.g., THF vs. hexane) and quenching protocols .
Q. How can researchers characterize the molecular structure and purity of this compound?
- Methodological Answer :
- Nuclear Magnetic Resonance (NMR) : ¹H and ¹³C NMR in deuterated chloroform (CDCl₃) confirm ligand coordination. For ²⁹Si NMR, a chemical shift of δ −10 to −15 ppm indicates tetrahedral coordination.
- Elemental Analysis : Carbon/nitrogen ratios should align with theoretical values (C: 47.1%, N: 27.4%).
- Mass Spectrometry (MS) : High-resolution MS (HRMS) should show a molecular ion peak at m/z 204.39 (C₈H₂₄N₄Si).
Discrepancies in crystallographic data (e.g., bond angles in X-ray studies) may arise from solvent adducts or lattice distortions, necessitating DFT calculations for validation .
Q. What are the stability considerations for this compound under ambient and experimental conditions?
- Methodological Answer : The compound hydrolyzes readily in humid air, forming silanol byproducts. Stability tests should include:
- Thermogravimetric Analysis (TGA) : Decomposition onset >150°C in inert atmospheres.
- Karl Fischer Titration : Moisture content <0.1% in stored samples.
- UV-Vis Spectroscopy : Monitor absorbance at 250–300 nm for degradation products. Contradictory reports on shelf life (3–6 months) highlight the need for rigorous argon-purged storage at −20°C .
Advanced Research Questions
Q. How can computational modeling resolve contradictions in the electronic structure of this compound?
- Methodological Answer : Density Functional Theory (DFT) simulations (e.g., B3LYP/6-311+G(d,p)) can predict molecular geometries, bond dissociation energies, and frontier orbital distributions. For example, discrepancies in Si–N bond lengths (1.70–1.75 Å in X-ray vs. 1.72 Å in DFT) may arise from crystal packing effects. Comparative studies with analogous compounds (e.g., Tetrakis(dimethylamino)silane) using Natural Bond Orbital (NBO) analysis clarify hyperconjugative interactions .
Q. What experimental designs are effective for studying the reactivity of this compound in cross-coupling reactions?
- Methodological Answer : Design a matrix of controlled variables:
- Catalyst Screening : Compare Pd(0) (e.g., Pd(PPh₃)₄) vs. Ni(0) catalysts in Suzuki-Miyaura couplings.
- Solvent Polarity : Test toluene (non-polar) vs. DMF (polar aprotic) to assess solvation effects.
- Substrate Scope : Evaluate aryl halides with varying steric/electronic profiles (e.g., 4-bromotoluene vs. 2-bromopyridine).
Contradictions in turnover numbers (TONs) often stem from trace oxygen or moisture, necessitating glovebox protocols .
Q. How should researchers address discrepancies in kinetic data for this compound-mediated reactions?
- Methodological Answer :
- Rate Law Determination : Use pseudo-first-order conditions with excess nucleophile (e.g., alcohol or amine) to isolate silane reactivity.
- Arrhenius Analysis : Compare activation energies (Eₐ) across temperature gradients (e.g., 25–80°C).
- In Situ Monitoring : Employ IR spectroscopy to track Si–N bond cleavage (∼950 cm⁻¹).
Conflicting Eₐ values (e.g., 50–65 kJ/mol) may reflect competing mechanistic pathways (associative vs. dissociative), resolved via isotopic labeling (²⁹Si or ¹⁵N) .
Q. Tables for Key Data
Properties
IUPAC Name |
N-ethyl-N-[tris(diethylamino)silyl]ethanamine | |
---|---|---|
Source | PubChem | |
URL | https://pubchem.ncbi.nlm.nih.gov | |
Description | Data deposited in or computed by PubChem | |
InChI |
InChI=1S/C16H40N4Si/c1-9-17(10-2)21(18(11-3)12-4,19(13-5)14-6)20(15-7)16-8/h9-16H2,1-8H3 | |
Source | PubChem | |
URL | https://pubchem.ncbi.nlm.nih.gov | |
Description | Data deposited in or computed by PubChem | |
InChI Key |
GURMJCMOXLWZHZ-UHFFFAOYSA-N | |
Source | PubChem | |
URL | https://pubchem.ncbi.nlm.nih.gov | |
Description | Data deposited in or computed by PubChem | |
Canonical SMILES |
CCN(CC)[Si](N(CC)CC)(N(CC)CC)N(CC)CC | |
Source | PubChem | |
URL | https://pubchem.ncbi.nlm.nih.gov | |
Description | Data deposited in or computed by PubChem | |
Molecular Formula |
C16H40N4Si | |
Source | PubChem | |
URL | https://pubchem.ncbi.nlm.nih.gov | |
Description | Data deposited in or computed by PubChem | |
DSSTOX Substance ID |
DTXSID6066153 | |
Record name | Silanetetramine, octaethyl- | |
Source | EPA DSSTox | |
URL | https://comptox.epa.gov/dashboard/DTXSID6066153 | |
Description | DSSTox provides a high quality public chemistry resource for supporting improved predictive toxicology. | |
Molecular Weight |
316.60 g/mol | |
Source | PubChem | |
URL | https://pubchem.ncbi.nlm.nih.gov | |
Description | Data deposited in or computed by PubChem | |
CAS No. |
17048-10-1 | |
Record name | N,N,N′,N′,N′′,N′′,N′′′,N′′′-Octaethylsilanetetramine | |
Source | CAS Common Chemistry | |
URL | https://commonchemistry.cas.org/detail?cas_rn=17048-10-1 | |
Description | CAS Common Chemistry is an open community resource for accessing chemical information. Nearly 500,000 chemical substances from CAS REGISTRY cover areas of community interest, including common and frequently regulated chemicals, and those relevant to high school and undergraduate chemistry classes. This chemical information, curated by our expert scientists, is provided in alignment with our mission as a division of the American Chemical Society. | |
Explanation | The data from CAS Common Chemistry is provided under a CC-BY-NC 4.0 license, unless otherwise stated. | |
Record name | Silanetetramine, N,N,N',N',N'',N'',N''',N'''-octaethyl- | |
Source | ChemIDplus | |
URL | https://pubchem.ncbi.nlm.nih.gov/substance/?source=chemidplus&sourceid=0017048101 | |
Description | ChemIDplus is a free, web search system that provides access to the structure and nomenclature authority files used for the identification of chemical substances cited in National Library of Medicine (NLM) databases, including the TOXNET system. | |
Record name | Silanetetramine, N,N,N',N',N'',N'',N''',N'''-octaethyl- | |
Source | EPA Chemicals under the TSCA | |
URL | https://www.epa.gov/chemicals-under-tsca | |
Description | EPA Chemicals under the Toxic Substances Control Act (TSCA) collection contains information on chemicals and their regulations under TSCA, including non-confidential content from the TSCA Chemical Substance Inventory and Chemical Data Reporting. | |
Record name | Silanetetramine, octaethyl- | |
Source | EPA DSSTox | |
URL | https://comptox.epa.gov/dashboard/DTXSID6066153 | |
Description | DSSTox provides a high quality public chemistry resource for supporting improved predictive toxicology. | |
Record name | Octaethylsilanetetramine | |
Source | European Chemicals Agency (ECHA) | |
URL | https://echa.europa.eu/substance-information/-/substanceinfo/100.037.363 | |
Description | The European Chemicals Agency (ECHA) is an agency of the European Union which is the driving force among regulatory authorities in implementing the EU's groundbreaking chemicals legislation for the benefit of human health and the environment as well as for innovation and competitiveness. | |
Explanation | Use of the information, documents and data from the ECHA website is subject to the terms and conditions of this Legal Notice, and subject to other binding limitations provided for under applicable law, the information, documents and data made available on the ECHA website may be reproduced, distributed and/or used, totally or in part, for non-commercial purposes provided that ECHA is acknowledged as the source: "Source: European Chemicals Agency, http://echa.europa.eu/". Such acknowledgement must be included in each copy of the material. ECHA permits and encourages organisations and individuals to create links to the ECHA website under the following cumulative conditions: Links can only be made to webpages that provide a link to the Legal Notice page. | |
Retrosynthesis Analysis
AI-Powered Synthesis Planning: Our tool employs the Template_relevance Pistachio, Template_relevance Bkms_metabolic, Template_relevance Pistachio_ringbreaker, Template_relevance Reaxys, Template_relevance Reaxys_biocatalysis model, leveraging a vast database of chemical reactions to predict feasible synthetic routes.
One-Step Synthesis Focus: Specifically designed for one-step synthesis, it provides concise and direct routes for your target compounds, streamlining the synthesis process.
Accurate Predictions: Utilizing the extensive PISTACHIO, BKMS_METABOLIC, PISTACHIO_RINGBREAKER, REAXYS, REAXYS_BIOCATALYSIS database, our tool offers high-accuracy predictions, reflecting the latest in chemical research and data.
Strategy Settings
Precursor scoring | Relevance Heuristic |
---|---|
Min. plausibility | 0.01 |
Model | Template_relevance |
Template Set | Pistachio/Bkms_metabolic/Pistachio_ringbreaker/Reaxys/Reaxys_biocatalysis |
Top-N result to add to graph | 6 |
Feasible Synthetic Routes
Disclaimer and Information on In-Vitro Research Products
Please be aware that all articles and product information presented on BenchChem are intended solely for informational purposes. The products available for purchase on BenchChem are specifically designed for in-vitro studies, which are conducted outside of living organisms. In-vitro studies, derived from the Latin term "in glass," involve experiments performed in controlled laboratory settings using cells or tissues. It is important to note that these products are not categorized as medicines or drugs, and they have not received approval from the FDA for the prevention, treatment, or cure of any medical condition, ailment, or disease. We must emphasize that any form of bodily introduction of these products into humans or animals is strictly prohibited by law. It is essential to adhere to these guidelines to ensure compliance with legal and ethical standards in research and experimentation.