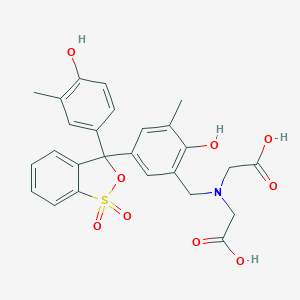
Semixylenol orange
Overview
Description
Semixylenol orange is a chemical compound with the molecular formula C26H25NO9S . It is an orange-yellow dye that is widely used in various scientific and industrial applications. This compound is known for its ability to form complexes with metal ions, making it a valuable reagent in analytical chemistry.
Preparation Methods
Synthetic Routes and Reaction Conditions
The preparation of semixylenol orange involves a one-step synthesis method. The process begins with the mixing of cresol red, iminodiacetic acid disodium salt, and glacial acetic acid. This mixture is heated to a temperature of 60 to 65 degrees Celsius until fully dissolved, then cooled to 25 to 30 degrees Celsius. A 37 percent formaldehyde solution is then added to the cooled mixture, which is allowed to rest for 7 to 8 hours and constantly stirred at 60 to 65 degrees Celsius. The unwanted solution is removed by decompression, and the remaining black thick matter is poured into an evaporation tank to be dried at 70 to 80 degrees Celsius, forming crystals. These crystals are then crushed to obtain the final orange-red solid product .
Industrial Production Methods
The one-step synthesizing method described above is suitable for large-scale industrial production. This method enhances the conversion rate and yields a product with high sensitivity, meeting the standards of similar products .
Chemical Reactions Analysis
Types of Reactions
Semixylenol orange undergoes various types of chemical reactions, including complexation with metal ions. It can form complexes with metals such as zinc, zirconium, and hafnium .
Common Reagents and Conditions
The complexation reactions typically involve the use of metal ions in acidic conditions. For example, the formation of complexes with zirconium (IV) and hafnium (IV) involves the use of perchloric acid .
Major Products Formed
Scientific Research Applications
Complexometric Titrations
SXO is widely recognized for its role as an indicator in complexometric titrations, particularly for determining metal ions such as thorium and zirconium.
- Thorium Determination : A method utilizing SXO as an indicator in the direct titration of thorium with ethylenediaminetetraacetic acid (EDTA) has been developed. The study highlighted the influence of various buffers on the titration process, demonstrating that SXO effectively indicates the endpoint due to its distinct color change in the presence of thorium ions .
- Zirconium Detection : Research has shown that an equimolar mixture of zirconium(IV) and SXO reacts rapidly with fluoride ions at low pH, resulting in a measurable increase in absorbance at approximately 530 nm. This reaction allows for sensitive detection of fluoride ions in various samples .
Spectrophotometric Applications
SXO's chromogenic properties make it suitable for spectrophotometric analyses, particularly in determining rare earth elements.
- Rare Earth Elements : A study examined the chromogenic reactions of SXO with rare earths and thorium, providing a reliable method for their spectrophotometric determination. The results indicated that SXO forms stable complexes with these elements, facilitating accurate quantification .
- Gadolinium and Terbium Microdetermination : Another application involves the spectrophotometric microdetermination of gadolinium(III) and terbium(III) using SXO. This method utilizes surfactants to enhance sensitivity and has been validated for practical use in analytical laboratories .
Environmental Monitoring
SXO can also be employed in environmental monitoring to detect and quantify pollutants.
- Fluoride Ion Detection : A colorimetric colony-screening assay using a zirconium-SXO chelate has been developed to detect fluoride ions released from fluorinated substrates. This method offers a rapid and effective approach to monitor fluoride levels in environmental samples .
Data Tables
Case Studies
- Determination of Thorium : A comprehensive study conducted by Oak Ridge National Laboratory demonstrated the efficacy of SXO in determining thorium concentrations in slurries. The research emphasized the importance of buffer selection to mitigate interference from other ions, showcasing SXO's adaptability in complex matrices .
- Spectrophotometric Analysis of Rare Earths : A detailed investigation into the chromogenic reactions between SXO and rare earth elements revealed that these complexes could be used for quantitative analysis in various industrial applications, providing essential data for resource management and environmental safety .
- Fluoride Detection Methodology : The development of a colorimetric assay utilizing zirconium-SXO chelates has provided a new tool for environmental scientists to monitor fluoride pollution effectively, highlighting SXO's role beyond traditional analytical chemistry applications .
Mechanism of Action
Semixylenol orange operates as a metallochromic indicator, forming complexes with various metal ions. It primarily targets metal ions such as zinc and zirconium, which play crucial roles in numerous biochemical processes, including enzymatic reactions and signal transduction . The rate of formation of these complexes is influenced by the dissociative interchange mechanism, involving the loss of coordinated water molecules at the metal ion sites .
Comparison with Similar Compounds
Similar Compounds
Xylenol orange: Another triphenylmethane dye used as a metallochromic indicator.
Cresol red: A pH indicator that is also used in the synthesis of semixylenol orange.
Iminodiacetic acid: A chelating agent used in the preparation of this compound.
Uniqueness
This compound is unique due to its high sensitivity and ability to form stable complexes with metal ions, making it a valuable reagent in analytical chemistry. Its versatility in various applications, including electrochemical processes and adsorptive removal studies, further distinguishes it from similar compounds.
Biological Activity
Semixylenol orange (SXO) is a synthetic compound derived from the condensation of o-cresol red, iminodiacetic acid, and formaldehyde. It is primarily recognized for its applications in analytical chemistry, particularly as a colorimetric reagent for the detection of metal ions. However, its biological activity has garnered attention in recent years, leading to investigations into its potential health benefits and mechanisms of action.
SXO is synthesized through a Mannich condensation process, resulting in a compound that exhibits distinct colorimetric properties. The purification of SXO can be achieved via high-performance liquid chromatography (HPLC), which allows for the separation of SXO from impurities and other by-products . Its chemical structure enables it to form complexes with various metal ions, making it useful in both analytical and biological contexts.
Antioxidant Properties
Research has indicated that SXO possesses significant antioxidant activity. Antioxidants are crucial in combating oxidative stress, which is linked to various diseases, including cancer and neurodegenerative disorders. SXO's ability to scavenge free radicals has been demonstrated in vitro, suggesting its potential role in reducing oxidative damage within biological systems .
Chelating Activity
SXO functions as a chelating agent, forming stable complexes with metal ions such as zirconium and alkaline-earth metals. This chelation can inhibit the catalytic activity of metal ions that contribute to oxidative stress and cellular damage. Studies have shown that SXO forms different complex species depending on the metal ion concentration and pH levels, which can influence its biological effects .
Case Studies
- Neuroprotective Effects : A study investigated the effects of SXO on neuronal cells exposed to oxidative stress. Results indicated that SXO treatment significantly reduced cell death and ROS production, enhancing cell viability compared to untreated controls. This suggests a protective mechanism against neurodegeneration .
- Metal Ion Interaction : In experiments assessing the interaction between SXO and various metal ions, it was found that SXO effectively reduced the cytotoxic effects associated with heavy metals by forming non-toxic complexes. This property highlights its potential use in detoxification therapies .
The biological activity of SXO can be attributed to several mechanisms:
- Radical Scavenging : SXO effectively neutralizes free radicals through electron donation, thereby mitigating oxidative damage.
- Metal Ion Chelation : By binding to harmful metal ions, SXO reduces their availability for catalyzing harmful reactions within cells.
- Regulation of Signaling Pathways : Preliminary studies suggest that SXO may influence signaling pathways associated with inflammation and apoptosis, further contributing to its protective effects against cellular stressors .
Data Summary
The following table summarizes key findings related to the biological activity of this compound:
Study | Cell Type/Model | Treatment Concentration | Outcome | Mechanism |
---|---|---|---|---|
Study 1 | Neuronal cells | 10-50 µM | ↑ Cell viability; ↓ ROS production | Antioxidant activity |
Study 2 | Heavy metal exposure | Variable | ↓ Cytotoxicity | Metal ion chelation |
Study 3 | In vitro models | 5-100 µM | ↑ Neuroprotection; ↓ apoptosis | Signaling pathway modulation |
Q & A
Basic Research Questions
Q. What is the role of semixylenol orange as a metallochromic indicator in trace metal analysis?
this compound forms stable complexes with metal ions like Bi³⁺, enabling colorimetric detection. In photometric methods (e.g., GB/T 223.48 for bismuth determination), it reacts with Bi³⁺ in acidic media (pH 1.5–2.5), producing a red complex with maximum absorbance at 535 nm. Methodological considerations include pH adjustment with nitric acid and masking interfering ions (e.g., Fe³⁺ with ascorbic acid) .
Q. How can researchers assess the purity and stability of this compound in laboratory settings?
Purity is evaluated via high-performance liquid chromatography (HPLC) with UV detection (λ = 440 nm) or titration against standardized bismuth solutions. Stability studies involve monitoring absorbance spectra over time under varying storage conditions (e.g., 4°C in amber vials to prevent photodegradation). Predictions of physical properties (e.g., density: 1.502 g/cm³, boiling point: 741.7°C) should be validated experimentally due to discrepancies in theoretical models .
Q. What are the standard protocols for preparing this compound solutions for spectrophotometric assays?
Dissolve 0.1 g of this compound in 100 mL of deionized water, acidify with 0.1 M HNO₃ to pH 2.0, and filter to remove particulates. Calibrate using Bi³⁺ standards (0.1–5.0 ppm) and validate linearity (R² > 0.995). Include blank corrections to account for matrix effects .
Advanced Research Questions
Q. How can researchers optimize this compound-based methods for detecting trace metals in complex matrices (e.g., biological fluids or alloys)?
Optimize pH (1.5–3.0 for Bi³⁺) and ionic strength to minimize interference. Use masking agents (e.g., EDTA for Cu²⁺, thiourea for Fe³⁺) and employ separation techniques like solid-phase extraction. Validate recovery rates (90–110%) via spike-and-recovery experiments and cross-check with ICP-MS .
Q. What experimental strategies address discrepancies in this compound’s molar absorptivity across studies?
Discrepancies arise from differences in pH, ionic strength, or instrumentation. Standardize conditions using NIST-traceable reference materials and report detailed metadata (e.g., spectrometer slit width, temperature). Perform interlaboratory comparisons to identify systematic errors .
Q. How does this compound’s performance compare to xylenol orange in metal-chelation assays?
this compound exhibits higher selectivity for Bi³⁺ and Sn⁴⁺ but lower sensitivity for Zr⁴⁺ compared to xylenol orange. Conduct comparative studies under identical conditions (pH, wavelength) and analyze limits of detection (LOD) and quantification (LOQ) using IUPAC guidelines .
Q. What are the implications of this compound’s photodegradation for long-term experiments?
Exposure to UV light reduces chromogenic activity. Design experiments with controlled lighting (e.g., amber glassware) and monitor degradation via periodic absorbance checks. For kinetic studies, use freshly prepared solutions and report storage durations .
Q. How can researchers validate novel applications of this compound in nanotechnology or environmental monitoring?
Characterize interactions with nanomaterials (e.g., Au nanoparticles) using TEM and dynamic light scattering. For environmental samples, validate against certified reference materials (e.g., NIST SRM 1640a) and assess matrix effects via standard addition methods .
Q. Methodological & Safety Considerations
Q. What steps ensure reproducibility when using this compound in collaborative studies?
Document all parameters: pH, temperature, reagent lot numbers, and instrument calibration data. Share raw spectra and statistical analyses (e.g., ANOVA for inter-group variability). Use collaborative platforms like Zenodo for data transparency .
Q. What safety protocols are critical when handling this compound in the laboratory?
Wear nitrile gloves, chemical-resistant goggles, and lab coats. Avoid inhalation of dust; use fume hoods for solution preparation. Store at room temperature away from oxidizers. In case of spills, absorb with inert material (e.g., vermiculite) and dispose as hazardous waste .
Properties
IUPAC Name |
2-[carboxymethyl-[[2-hydroxy-5-[3-(4-hydroxy-3-methylphenyl)-1,1-dioxo-2,1λ6-benzoxathiol-3-yl]-3-methylphenyl]methyl]amino]acetic acid | |
---|---|---|
Source | PubChem | |
URL | https://pubchem.ncbi.nlm.nih.gov | |
Description | Data deposited in or computed by PubChem | |
InChI |
InChI=1S/C26H25NO9S/c1-15-9-18(7-8-21(15)28)26(20-5-3-4-6-22(20)37(34,35)36-26)19-10-16(2)25(33)17(11-19)12-27(13-23(29)30)14-24(31)32/h3-11,28,33H,12-14H2,1-2H3,(H,29,30)(H,31,32) | |
Source | PubChem | |
URL | https://pubchem.ncbi.nlm.nih.gov | |
Description | Data deposited in or computed by PubChem | |
InChI Key |
OGODTFKKGYRAQJ-UHFFFAOYSA-N | |
Source | PubChem | |
URL | https://pubchem.ncbi.nlm.nih.gov | |
Description | Data deposited in or computed by PubChem | |
Canonical SMILES |
CC1=CC(=CC(=C1O)CN(CC(=O)O)CC(=O)O)C2(C3=CC=CC=C3S(=O)(=O)O2)C4=CC(=C(C=C4)O)C | |
Source | PubChem | |
URL | https://pubchem.ncbi.nlm.nih.gov | |
Description | Data deposited in or computed by PubChem | |
Molecular Formula |
C26H25NO9S | |
Source | PubChem | |
URL | https://pubchem.ncbi.nlm.nih.gov | |
Description | Data deposited in or computed by PubChem | |
DSSTOX Substance ID |
DTXSID20941002 | |
Record name | 2,2'-[({2-Hydroxy-5-[3-(4-hydroxy-3-methylphenyl)-1,1-dioxo-1,3-dihydro-2,1lambda~6~-benzoxathiol-3-yl]-3-methylphenyl}methyl)azanediyl]diacetic acid | |
Source | EPA DSSTox | |
URL | https://comptox.epa.gov/dashboard/DTXSID20941002 | |
Description | DSSTox provides a high quality public chemistry resource for supporting improved predictive toxicology. | |
Molecular Weight |
527.5 g/mol | |
Source | PubChem | |
URL | https://pubchem.ncbi.nlm.nih.gov | |
Description | Data deposited in or computed by PubChem | |
CAS No. |
19329-67-0 | |
Record name | Semi-xylenol orange | |
Source | ChemIDplus | |
URL | https://pubchem.ncbi.nlm.nih.gov/substance/?source=chemidplus&sourceid=0019329670 | |
Description | ChemIDplus is a free, web search system that provides access to the structure and nomenclature authority files used for the identification of chemical substances cited in National Library of Medicine (NLM) databases, including the TOXNET system. | |
Record name | 2,2'-[({2-Hydroxy-5-[3-(4-hydroxy-3-methylphenyl)-1,1-dioxo-1,3-dihydro-2,1lambda~6~-benzoxathiol-3-yl]-3-methylphenyl}methyl)azanediyl]diacetic acid | |
Source | EPA DSSTox | |
URL | https://comptox.epa.gov/dashboard/DTXSID20941002 | |
Description | DSSTox provides a high quality public chemistry resource for supporting improved predictive toxicology. | |
Retrosynthesis Analysis
AI-Powered Synthesis Planning: Our tool employs the Template_relevance Pistachio, Template_relevance Bkms_metabolic, Template_relevance Pistachio_ringbreaker, Template_relevance Reaxys, Template_relevance Reaxys_biocatalysis model, leveraging a vast database of chemical reactions to predict feasible synthetic routes.
One-Step Synthesis Focus: Specifically designed for one-step synthesis, it provides concise and direct routes for your target compounds, streamlining the synthesis process.
Accurate Predictions: Utilizing the extensive PISTACHIO, BKMS_METABOLIC, PISTACHIO_RINGBREAKER, REAXYS, REAXYS_BIOCATALYSIS database, our tool offers high-accuracy predictions, reflecting the latest in chemical research and data.
Strategy Settings
Precursor scoring | Relevance Heuristic |
---|---|
Min. plausibility | 0.01 |
Model | Template_relevance |
Template Set | Pistachio/Bkms_metabolic/Pistachio_ringbreaker/Reaxys/Reaxys_biocatalysis |
Top-N result to add to graph | 6 |
Feasible Synthetic Routes
Disclaimer and Information on In-Vitro Research Products
Please be aware that all articles and product information presented on BenchChem are intended solely for informational purposes. The products available for purchase on BenchChem are specifically designed for in-vitro studies, which are conducted outside of living organisms. In-vitro studies, derived from the Latin term "in glass," involve experiments performed in controlled laboratory settings using cells or tissues. It is important to note that these products are not categorized as medicines or drugs, and they have not received approval from the FDA for the prevention, treatment, or cure of any medical condition, ailment, or disease. We must emphasize that any form of bodily introduction of these products into humans or animals is strictly prohibited by law. It is essential to adhere to these guidelines to ensure compliance with legal and ethical standards in research and experimentation.