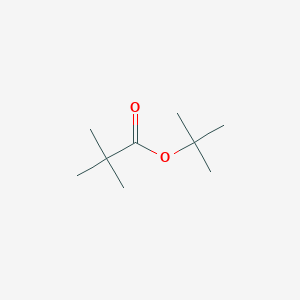
Tert-butyl pivalate
Overview
Description
Tert-butyl pivalate (CAS: 16474-43-4), also known as tert-butyl trimethylacetate, is an ester derived from pivalic acid (2,2-dimethylpropanoic acid) and tert-butanol. Its molecular formula is C₉H₁₈O₂, and its structure features two bulky tert-butyl groups (one from the pivalate moiety and another from the tert-butanol), which significantly influence its chemical and physical properties . This compound is a colorless liquid with a fruity odor and is utilized in diverse applications, including pharmaceuticals, organic synthesis, and as a solvent . Its steric bulk and resistance to hydrolysis make it particularly valuable in reactions requiring stability under harsh conditions .
Preparation Methods
Synthetic Routes and Reaction Conditions: Tert-butyl pivalate can be synthesized through the esterification of pivalic acid with tert-butyl alcohol. This reaction typically requires an acid catalyst such as sulfuric acid to proceed efficiently. The reaction can be represented as follows:
(CH3)3CCO2H+(CH3)3COH→(CH3)3CCO2C(CH3)3+H2O
In this reaction, pivalic acid reacts with tert-butyl alcohol to form this compound and water.
Industrial Production Methods: On an industrial scale, this compound can be produced using similar esterification methods. The process involves the use of large-scale reactors and continuous distillation to separate the ester from the reaction mixture. The use of catalysts such as sulfuric acid or phosphoric acid can enhance the reaction rate and yield .
Chemical Reactions Analysis
Types of Reactions: Tert-butyl pivalate undergoes various chemical reactions, including:
Hydrolysis: In the presence of strong acids or bases, this compound can hydrolyze to form pivalic acid and tert-butyl alcohol.
Reduction: It can be reduced to form the corresponding alcohols using reducing agents like lithium aluminum hydride.
Substitution: The ester group can be substituted by nucleophiles such as amines or alcohols under appropriate conditions.
Common Reagents and Conditions:
Hydrolysis: Strong acids (e.g., hydrochloric acid) or bases (e.g., sodium hydroxide) at elevated temperatures.
Reduction: Lithium aluminum hydride in anhydrous ether.
Substitution: Nucleophiles such as amines or alcohols in the presence of a base like pyridine.
Major Products Formed:
Hydrolysis: Pivalic acid and tert-butyl alcohol.
Reduction: Corresponding alcohols.
Substitution: Corresponding amides or esters depending on the nucleophile used.
Scientific Research Applications
Green Chemistry Applications
TBP has emerged as a promising alternative solvent in green chemistry due to its low volatility and reduced environmental impact compared to conventional solvents like toluene.
- Solvent Replacement : TBP has been identified as a suitable candidate for replacing hazardous solvents in processes such as the dissolution of natural rubber and other polymers. In a study evaluating various esters, TBP demonstrated effective solubility properties while maintaining a lower environmental footprint .
Property | Tert-butyl Pivalate | Toluene |
---|---|---|
Boiling Point (°C) | 134 | 110 |
Polarity | Low | Moderate |
Environmental Impact | Lower | Higher |
- Polymerization Initiator : TBP is also utilized in polymerization processes as a solvent or co-solvent. Its properties facilitate reactions at lower temperatures, which can enhance product quality and reduce energy consumption .
Pharmaceutical Applications
In the pharmaceutical industry, TBP serves as an important intermediate in the synthesis of prodrugs and other pharmaceutical compounds.
- Prodrug Development : TBP is used to enhance the bioavailability of certain drugs. For instance, it has been involved in synthesizing prodrugs that improve the pharmacokinetic profiles of active pharmaceutical ingredients (APIs) by modifying their solubility and permeability across biological membranes .
- Case Study : A recent study highlighted the synthesis of DPTIP prodrugs using TBP, which significantly improved oral bioavailability and brain penetration compared to the parent compound. This demonstrates TBP's role in optimizing drug delivery systems .
Polymer Synthesis
TBP is used as an initiator or solvent in the synthesis of various polymers.
- Poly(vinyl alcohol) Production : Research indicates that TBP can be used to derive highly isotactic poly(vinyl alcohol) from tert-butyl vinyl ether. This application showcases TBP’s utility in producing high-performance materials with desirable mechanical properties .
- Continuous Synthesis : Innovative methods have been developed for the continuous synthesis of TBP-based compounds using microreactor technology, enhancing efficiency and scalability in polymer production .
Industrial Applications
In industrial settings, TBP's properties make it suitable for various applications beyond pharmaceuticals and green chemistry.
- Chemical Manufacturing : TBP is employed in the manufacturing of specialty chemicals where its unique solvent properties can be leveraged to optimize reaction conditions and product yields.
- Safety Profile : While TBP is flammable and reactive, its controlled use within established safety protocols mitigates risks associated with its application in industrial processes .
Mechanism of Action
The mechanism of action of tert-butyl pivalate primarily involves its role as an ester. In chemical reactions, it can act as a substrate for hydrolysis, reduction, or substitution reactions. The molecular targets and pathways involved depend on the specific reaction and conditions used. For example, in hydrolysis, the ester bond is cleaved by nucleophilic attack from water or hydroxide ions, leading to the formation of pivalic acid and tert-butyl alcohol .
Comparison with Similar Compounds
Stability and Hydrolysis Resistance
Tert-butyl pivalate exhibits exceptional resistance to hydrolysis compared to other esters. For example:
- Tert-butyl crotonate and tert-butyl cyclohexanecarboxylate are prone to hydrolysis in the absence of alcohols, forming anhydrides or decomposing under acidic conditions .
- In contrast, the this compound group remains intact even in biological environments, as demonstrated by its use in prodrugs designed to cross the blood-brain barrier (e.g., resveratrol derivatives like PIV) . This stability arises from the steric hindrance of the tert-butyl groups, which shield the ester bond from nucleophilic attack .
Table 1: Hydrolysis Resistance of Selected Esters
Solubility and Solvent Properties
The bulky tert-butyl groups in this compound enhance its solubility in nonpolar solvents, making it superior to carboxylates like stearate in applications requiring compatibility with hydrophobic matrices (e.g., single-molecule magnets) . In contrast:
- Tert-butyl acetate and sec-butyl acetate are more polar due to shorter alkyl chains, limiting their use in nonpolar systems .
- Methyl pivalate (CAS: 5129-38-4), though structurally similar, has a lower molecular weight (C₈H₁₆O₂), resulting in a lower boiling point (~140°C vs. ~160°C for this compound) and reduced steric bulk .
Table 2: Physical Properties of Pivalate Derivatives
Compound | Boiling Point (°C) | Solubility in Nonpolar Solvents | Molecular Weight |
---|---|---|---|
This compound | ~160 | Excellent | 158.24 |
Methyl pivalate | ~140 | Good | 144.21 |
Tert-butyl acetate | ~98 | Moderate | 116.16 |
Ethyl isobutyrate | ~110 | Poor | 116.16 |
Table 3: Reaction Efficiency of Esters in Catalytic Processes
Reaction Type | Ester Used | Yield/Selectivity | Key Advantage |
---|---|---|---|
Allylation (Pd catalysis) | This compound | 15% yield, >19:1 dr | Enhanced electrophile stability |
ROAC polymerization | This compound | No chain transfer | Suppressed pivalate nucleophilicity |
Biological Activity
Tert-butyl pivalate (TBP), a compound with the chemical formula CHO, is an ester formed from tert-butyl alcohol and pivalic acid. This compound has garnered attention in various fields, including medicinal chemistry and organic synthesis, due to its unique structural properties and biological activities. This article delves into the biological activity of TBP, highlighting its synthesis, metabolic stability, and potential applications in drug development.
Chemical Structure and Properties
This compound is characterized by its bulky tert-butyl group, which contributes to its steric properties. The structure can be represented as follows:
This compound exhibits significant lipophilicity due to the presence of the tert-butyl group, which can influence its biological interactions.
Synthesis of this compound
The synthesis of this compound typically involves the esterification of pivalic acid with tert-butyl alcohol. The reaction can be summarized as follows:
- Reactants : Pivalic acid and tert-butyl alcohol.
- Catalyst : Acid catalyst (e.g., sulfuric acid).
- Reaction Conditions : Heating under reflux to promote ester formation.
The reaction yields this compound along with water as a byproduct.
1. Metabolic Stability
One of the key aspects of TBP's biological activity is its metabolic stability. Research indicates that compounds containing tert-butyl groups often exhibit high metabolic lability due to oxidative metabolism, primarily involving cytochrome P450 enzymes . However, modifications to the structure, such as replacing hydrogen atoms with fluorine, have been shown to enhance metabolic stability without significantly affecting biological activity .
In vitro studies have demonstrated that TBP undergoes oxidative metabolism predominantly at the tert-butyl group, leading to various metabolites that may exhibit different biological effects . This metabolic pathway is crucial for understanding the pharmacokinetics of TBP in therapeutic applications.
2. Cytotoxicity and Antimicrobial Properties
This compound has been investigated for its cytotoxic properties, particularly in relation to natural products derived from marine organisms. For instance, apratoxin A, a compound produced by the cyanobacterium Moorea bouillonii, contains a pivaloyl moiety that contributes to its cytotoxic activity against certain cancer cell lines . The biosynthetic pathway of apratoxin A reveals that the formation of pivaloyl-ACP is catalyzed by a bifunctional enzyme that performs both decarboxylation and methylation reactions .
Additionally, TBP has been evaluated for its potential antimicrobial properties. In studies involving various bacterial strains, TBP exhibited inhibitory effects, suggesting its utility as a lead compound for developing new antimicrobial agents .
Case Study 1: Synthesis and Biological Evaluation
A study explored the synthesis of solasodine pivalate from diosgenin pivalate using tert-butyl carbamate as a reagent. The findings indicated that steric hindrance from the bulky tert-butyl group resulted in low substrate conversion during reactions . However, optimizing reaction conditions improved yields significantly, demonstrating the importance of structural considerations in synthetic pathways involving TBP.
Case Study 2: Metabolic Stability Comparison
A comparative study assessed the metabolic stability of TBP against analogs containing trifluoromethyl groups. Results showed that substituting the tert-butyl group with a trifluoromethylcyclopropyl group led to enhanced metabolic stability both in vitro and in vivo . This finding underscores the relevance of structural modifications in improving drug-like properties while retaining biological efficacy.
Q & A
Q. What experimental protocols are recommended for synthesizing tert-butyl pivalate, and how can purity be validated?
Basic Research Question
tert-Butyl pivalate is typically synthesized via esterification reactions between pivalic acid and tert-butanol under acid catalysis. Methodological considerations include:
- Catalyst selection : Sulfuric acid or p-toluenesulfonic acid are common catalysts, but anhydrous conditions are critical to avoid side reactions like hydrolysis .
- Purification : Distillation under reduced pressure (e.g., 40–60 °C at 10–15 mmHg) followed by column chromatography (silica gel, hexane/ethyl acetate) ensures high purity (>98%) .
- Validation : Confirm purity via gas chromatography (GC) with flame ionization detection (FID) and nuclear magnetic resonance (NMR) spectroscopy. Key spectral markers include a singlet for the tert-butyl group (δ 1.2 ppm in H NMR) and carbonyl resonance (δ 170–175 ppm in C NMR) .
Q. How do temperature and solvent systems influence the decomposition pathways of this compound?
Advanced Research Question
Decomposition studies reveal competing pathways:
- Primary pathway : Thermal cleavage at 120–150 °C yields carbon dioxide, pivalic anhydride, and tert-butanol (88% via β-scission) .
- Secondary pathway : Radical-mediated decomposition produces di-tert-butyl carbonate and isobutene (12%) under inert atmospheres .
Methodological Recommendations : - Use variable-temperature GC-mass spectrometry (GC-MS) to track volatile products.
- Monitor non-volatile residues via H NMR and infrared (IR) spectroscopy for anhydride formation.
- Compare product ratios (e.g., pivalic anhydride/tert-butyl pivalate = 3.7:1) to validate mechanistic dominance .
Q. What structure-activity relationships (SAR) govern the antitumor potential of this compound derivatives?
Advanced Research Question
SAR studies on ester derivatives suggest:
- Ester chain length : Linear two-carbon chains (e.g., ethyl esters) enhance chemosensitizing activity, while longer chains (>5 carbons) reduce efficacy .
- Bulkier substituents : tert-Butyl groups (as in tert-butyl pivalate) improve metabolic stability but may hinder target binding due to steric effects .
Experimental Design : - Synthesize analogs with systematic substituent variations (e.g., isopropyl, neopentyl).
- Evaluate cytotoxicity in tumor cell lines (e.g., MCF-7, HepG2) and compare survival rates (e.g., 13–14% for optimal derivatives) .
Q. How can contradictions in catalytic efficiency of this compound in cross-coupling reactions be resolved?
Advanced Research Question
Discrepancies in palladium-catalyzed allylation (e.g., low yields vs. high enantioselectivity) arise from:
- Electrophile stability : tert-Butyl pivalate esters resist decomposition under basic conditions, unlike carbonate analogs .
- Reaction optimization : Adjusting ligands (e.g., chiral phosphines) and solvents (e.g., THF vs. DMF) improves yields (e.g., from 15% to >50%) while retaining stereoselectivity (>19:1 dr, 95% ee) .
Methodological Steps : - Conduct kinetic studies to identify rate-limiting steps.
- Use high-throughput screening for ligand/solvent combinations.
Q. What spectroscopic and chromatographic techniques are essential for characterizing this compound in complex mixtures?
Basic Research Question
- GC-MS : Resolves volatile decomposition products (e.g., isobutene, CO₂) with electron ionization (EI) for fragmentation patterns .
- HPLC-DAD : Quantifies non-volatile byproducts (e.g., pivalic anhydride) using C18 columns and UV detection at 210 nm .
- 2D NMR (HSQC, HMBC) : Assigns quaternary carbons and confirms ester linkage integrity .
Q. How should researchers address discrepancies in reported thermodynamic stability data for this compound?
Advanced Research Question
Conflicting stability data (e.g., decomposition temperatures) may stem from:
- Impurity effects : Trace acids or moisture accelerate degradation. Validate reagent purity via Karl Fischer titration .
- Experimental conditions : Differential scanning calorimetry (DSC) under nitrogen vs. air alters activation energies. Standardize inert-atmosphere protocols .
Resolution Strategy : - Replicate studies using identical instrumentation (e.g., same GC column, temperature ramp rates).
- Publish raw DSC thermograms and kinetic data for peer validation .
Properties
IUPAC Name |
tert-butyl 2,2-dimethylpropanoate | |
---|---|---|
Source | PubChem | |
URL | https://pubchem.ncbi.nlm.nih.gov | |
Description | Data deposited in or computed by PubChem | |
InChI |
InChI=1S/C9H18O2/c1-8(2,3)7(10)11-9(4,5)6/h1-6H3 | |
Source | PubChem | |
URL | https://pubchem.ncbi.nlm.nih.gov | |
Description | Data deposited in or computed by PubChem | |
InChI Key |
VXHFNALHLRWIIU-UHFFFAOYSA-N | |
Source | PubChem | |
URL | https://pubchem.ncbi.nlm.nih.gov | |
Description | Data deposited in or computed by PubChem | |
Canonical SMILES |
CC(C)(C)C(=O)OC(C)(C)C | |
Source | PubChem | |
URL | https://pubchem.ncbi.nlm.nih.gov | |
Description | Data deposited in or computed by PubChem | |
Molecular Formula |
C9H18O2 | |
Source | PubChem | |
URL | https://pubchem.ncbi.nlm.nih.gov | |
Description | Data deposited in or computed by PubChem | |
DSSTOX Substance ID |
DTXSID60333947 | |
Record name | tert-butyl pivalate | |
Source | EPA DSSTox | |
URL | https://comptox.epa.gov/dashboard/DTXSID60333947 | |
Description | DSSTox provides a high quality public chemistry resource for supporting improved predictive toxicology. | |
Molecular Weight |
158.24 g/mol | |
Source | PubChem | |
URL | https://pubchem.ncbi.nlm.nih.gov | |
Description | Data deposited in or computed by PubChem | |
CAS No. |
16474-43-4 | |
Record name | tert-butyl pivalate | |
Source | EPA DSSTox | |
URL | https://comptox.epa.gov/dashboard/DTXSID60333947 | |
Description | DSSTox provides a high quality public chemistry resource for supporting improved predictive toxicology. | |
Retrosynthesis Analysis
AI-Powered Synthesis Planning: Our tool employs the Template_relevance Pistachio, Template_relevance Bkms_metabolic, Template_relevance Pistachio_ringbreaker, Template_relevance Reaxys, Template_relevance Reaxys_biocatalysis model, leveraging a vast database of chemical reactions to predict feasible synthetic routes.
One-Step Synthesis Focus: Specifically designed for one-step synthesis, it provides concise and direct routes for your target compounds, streamlining the synthesis process.
Accurate Predictions: Utilizing the extensive PISTACHIO, BKMS_METABOLIC, PISTACHIO_RINGBREAKER, REAXYS, REAXYS_BIOCATALYSIS database, our tool offers high-accuracy predictions, reflecting the latest in chemical research and data.
Strategy Settings
Precursor scoring | Relevance Heuristic |
---|---|
Min. plausibility | 0.01 |
Model | Template_relevance |
Template Set | Pistachio/Bkms_metabolic/Pistachio_ringbreaker/Reaxys/Reaxys_biocatalysis |
Top-N result to add to graph | 6 |
Feasible Synthetic Routes
Disclaimer and Information on In-Vitro Research Products
Please be aware that all articles and product information presented on BenchChem are intended solely for informational purposes. The products available for purchase on BenchChem are specifically designed for in-vitro studies, which are conducted outside of living organisms. In-vitro studies, derived from the Latin term "in glass," involve experiments performed in controlled laboratory settings using cells or tissues. It is important to note that these products are not categorized as medicines or drugs, and they have not received approval from the FDA for the prevention, treatment, or cure of any medical condition, ailment, or disease. We must emphasize that any form of bodily introduction of these products into humans or animals is strictly prohibited by law. It is essential to adhere to these guidelines to ensure compliance with legal and ethical standards in research and experimentation.