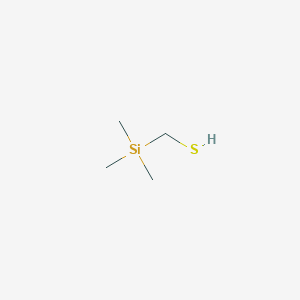
Trimethylsilylmethanethiol
Overview
Description
Trimethylsilylmethanethiol (CAS: 18165-76-9), with the molecular formula C₄H₁₂SSi and molecular weight 120.29 g/mol, is a silicon-containing organosulfur compound. It features a trimethylsilyl group (-Si(CH₃)₃) attached to a methanethiol (-CH₂SH) backbone. Key physical properties include a density of 0.8 g/cm³, boiling point of 113.1°C at 760 mmHg, and a flash point of 22.2°C, classifying it as a highly flammable liquid .
Its GHS classification highlights hazards such as flammability (Category 2), skin irritation (Category 2), and serious eye irritation (Category 2A) . The compound is primarily used in organic synthesis as a thiol-protecting group due to the stability of the trimethylsilyl moiety under acidic or basic conditions .
Preparation Methods
Synthetic Routes and Reaction Conditions: Trimethylsilylmethanethiol can be synthesized through the reaction of trimethylchlorosilane with sodium hydrosulfide. The reaction typically occurs in an inert atmosphere to prevent oxidation and moisture interference. The general reaction is as follows:
(CH3)3SiCl+NaSH→(CH3)3SiCH2SH+NaCl
Industrial Production Methods: In industrial settings, the production of this compound involves similar synthetic routes but on a larger scale. The reaction conditions are optimized for higher yields and purity. The process includes careful control of temperature, pressure, and the use of high-purity reagents to ensure consistent product quality .
Chemical Reactions Analysis
Oxidation Reactions
TMS-SH undergoes oxidation to form disulfides and sulfonates, critical for interfacial stabilization in electrochemical systems .
Mechanism :
-
Disulfide Formation :
Oxidation with iodine or atmospheric oxygen generates the disulfide derivative:
This reaction is pivotal in forming protective cathode-electrolyte interphases (CEI) in lithium-ion batteries, enhancing cycle stability . -
Sulfonate Formation :
Strong oxidizing agents like hydrogen peroxide further oxidize disulfides to sulfonic acids:
Reagents and Conditions :
Reaction Type | Reagents | Conditions | Major Product |
---|---|---|---|
Disulfide formation | I, O | RT, aerobic | Bis(2-(trimethylsilyl)ethyl)disulfide |
Sulfonate formation | HO, HNO | 60–80°C, acidic medium | 2-(Trimethylsilyl)ethanesulfonic acid |
Substitution Reactions
The thiol group in TMS-SH participates in nucleophilic substitutions, forming thioethers or sulfides .
Mechanism :
-
SN_\text{N}N2 Pathway :
TMS-SH reacts with alkyl halides (e.g., 1-bromobutane) in the presence of a base:
This reaction is facilitated by polar aprotic solvents like DMF . -
β-Silyl Carbocation Intermediate :
In acidic media, TMS-SH generates a stabilized β-silyl carbocation, which reacts with nucleophiles (e.g., carboxylates) :
Applications :
-
Esterification of carboxylic acids under mild conditions (e.g., 4-methoxybenzoic acid → 4-methoxybenzoate ester) .
Addition Reactions
TMS-SH undergoes electrophilic additions, forming sulfenyl chlorides or thiiranium ions .
Mechanism :
-
Electrophilic Addition :
Reaction with chlorine or sulfuryl chloride yields sulfenyl chlorides:
-
Thiol-Ene Reaction :
Under blue-light irradiation, TMS-SH adds across alkenes via a radical mechanism :
Reaction Conditions :
Substrate | Reagent/Catalyst | Product | Yield (%) |
---|---|---|---|
Styrene | TMS-SH, acridine orange | β-Hydroxysulfide | 82 |
Cyclohexene | Cl | 2-(Trimethylsilyl)ethyl sulfenyl chloride | 75 |
Cross-Coupling Reactions
TMS-SH participates in silicon-based cross-couplings, enabling C–S bond formation in complex syntheses .
Mechanism :
-
Hydrosilylation/Palladium Catalysis :
TMS-SH reacts with alkynes in the presence of Pt or Pd catalysts, followed by fluoride-mediated coupling:
Applications :
Scientific Research Applications
Organic Synthesis
Trimethylsilylmethanethiol is utilized as a reagent in organic synthesis, particularly in the formation of silylated compounds. It serves as a protecting group for thiols, enhancing selectivity in chemical reactions. This property is crucial for synthesizing complex organic molecules where thiol functionalities are involved.
Electrochemical Applications
Recent studies have identified trimethylsilyl compounds, including this compound, as solid electrolyte additives in all-solid-state batteries (ASSBs). These compounds improve interfacial stability between sulfide-based solid electrolytes and cathode materials. Specifically, 2-(trimethylsilyl)ethanethiol (a related compound) has been shown to form stable cathode-electrolyte interphase (CEI) layers that suppress interfacial degradation during cycling. This leads to enhanced electrochemical performance, such as superior cycle life retention after extensive charge-discharge cycles .
Application Area | Specific Use Case | Benefits |
---|---|---|
Organic Synthesis | Reagent for silylation | Enhances selectivity and stability |
Battery Technology | Solid electrolyte additive | Improves electrochemical performance |
Surface Chemistry | Modification of surfaces | Enhances adhesion and stability |
Biological Applications
In biological research, this compound is employed in the modification of biomolecules and studying thiol-based redox processes. Its ability to form strong bonds with metals makes it useful in bioconjugation techniques where thiol groups are involved.
Medicinal Chemistry
The compound is being investigated for its potential use in drug development. Its reactivity allows for the creation of novel pharmaceutical intermediates, particularly those involving thiol functionalities . Research into its medicinal properties is ongoing, focusing on its applications in targeted drug delivery systems.
Industrial Applications
In industry, this compound is used in producing specialty chemicals and materials, including polymers and coatings. Its properties enhance the durability and performance of these materials under various conditions .
Case Study 1: Battery Performance Enhancement
A study conducted on all-solid-state batteries demonstrated that incorporating trimethylsilyl compounds significantly improved the interfacial stability between lithium sulfide-based electrolytes and cathode materials like LiCoO. The CEI layer formed from these compounds led to an impressive capacity retention of 85% after 2000 cycles at 30°C .
Case Study 2: Bioconjugation Techniques
Research into bioconjugation using this compound has shown promising results in modifying proteins for targeted drug delivery. The compound's reactivity with thiols facilitates the attachment of therapeutic agents to biomolecules, enhancing their efficacy while minimizing side effects .
Mechanism of Action
The mechanism of action of trimethylsilylmethanethiol involves the reactivity of the thiol group. The thiol group can form strong bonds with metals and other electrophiles, making it a versatile reagent in various chemical reactions. The silyl group provides steric protection and enhances the stability of the thiol group, allowing for selective reactions. The molecular targets and pathways involved include thiol-disulfide exchange reactions and radical-based mechanisms .
Comparison with Similar Compounds
Trimethylsilylmethanethiol is structurally and functionally distinct from other silyl- or aryl-substituted thiols. Below is a detailed comparison:
Structural and Physical Properties
Compound Name | Molecular Formula | Molecular Weight (g/mol) | Boiling Point (°C) | Density (g/cm³) | Key Structural Features |
---|---|---|---|---|---|
This compound | C₄H₁₂SSi | 120.29 | 113.1 | 0.8 | Si(CH₃)₃-CH₂SH; compact silyl group |
3-(Trimethoxysilyl)-1-propanethiol | C₆H₁₆O₃SSi | 212.37 | N/A | N/A | (CH₃O)₃Si-(CH₂)₃SH; hydrolytically active |
Trifluoromethanethiol | CF₃SH | 102.08 | ~-20 (estimated) | 1.3 (estimated) | CF₃-SH; high electronegativity |
Triphenylmethanethiol | C₁₉H₁₆S | 276.40 | N/A | N/A | (C₆H₅)₃C-SH; bulky trityl group |
Notes:
- This compound has a lower molecular weight and higher volatility compared to aryl-substituted thiols like Triphenylmethanethiol .
- 3-(Trimethoxysilyl)-1-propanethiol ’s trimethoxy group increases its susceptibility to hydrolysis, making it suitable for crosslinking applications .
- Trifluoromethanethiol ’s boiling point is significantly lower due to the small molecular size and fluorine’s volatility .
Key Observations :
Biological Activity
Trimethylsilylmethanethiol, also known as 2-(Trimethylsilyl)ethanethiol (TMS-SH), is an organosilicon compound characterized by a thiol (-SH) group bonded to a trimethylsilyl group. This compound has garnered attention for its unique chemical properties and potential biological activities. This article explores its biological activity, mechanisms of action, and applications based on diverse research findings.
- Molecular Formula : C5H14SSi
- Appearance : Colorless liquid with a distinct odor
- Reactivity : Functions primarily as a protecting group in biochemical syntheses and exhibits reactivity typical of thiols, including oxidation and nucleophilic substitution.
This compound acts primarily as a protecting reagent for carboxyl and phosphate groups during chemical reactions. By forming stable silyl sulfides, it enhances the stability and reactivity of various substrates, making it valuable in synthetic chemistry and biological applications.
Antimicrobial Properties
Research indicates that TMS-SH and its derivatives exhibit antimicrobial properties, likely due to the reactivity of the thiol group. Thiols are known to interact with various biological systems, which can lead to the inhibition of microbial growth. This property is particularly relevant in medicinal chemistry where TMS-SH is investigated for its potential in developing antimicrobial agents.
Interaction with Biological Systems
While specific biological mechanisms of action for TMS-SH remain underexplored, studies suggest that its derivatives may influence nucleic acid metabolism. For instance, thionucleosides synthesized from TMS-SH have shown potential therapeutic applications. Moreover, the compound's ability to stabilize lipid peroxidation products indicates a role in cellular protection against oxidative stress .
Case Studies
- Esterification Reactions : A study demonstrated that 2-(Trimethylsilyl)ethanethiol could be used effectively in esterification reactions without requiring additional catalysts. This finding underscores its utility in organic synthesis and potential pharmaceutical applications .
- Electrochemical Applications : In battery technology, TMS-SH was shown to improve the interfacial stability between sulfide-based solid electrolytes and cathode materials. This enhancement leads to superior electrochemical performance, highlighting its relevance in energy storage systems .
Stability and Reactivity
- Oxidation Reactions : TMS-SH can be oxidized to form disulfides or sulfonates, which are important intermediates in various chemical processes. These reactions can be catalyzed by iodine or hydrogen peroxide under controlled conditions.
- Nucleophilic Substitution : The thiol group can participate in nucleophilic substitution reactions, allowing for further functionalization of organic molecules.
Comparative Analysis
To understand the uniqueness of TMS-SH compared to other thiols, the following table summarizes key features:
Compound Name | Structure | Key Features |
---|---|---|
Ethanethiol | CH₃CH₂SH | Simple thiol; strong odor; used in organic synthesis |
Trimethylsilylthiocyanate | CH₃₃SiNCS | Contains a thiocyanate group; used in various syntheses |
3-Mercaptopropionic acid | HSCH₂CH₂COOH | Contains a carboxylic acid group; important in biochemistry |
2-Mercaptoethanol | HOCH₂CH₂SH | Commonly used as a reducing agent; similar reactivity profile |
2-(Trimethylsilyl)ethanethiol | (CH₃)₃SiCH₂CH₂SH | Enhanced stability and reactivity due to trimethylsilyl group |
Q & A
Basic Research Questions
Q. What are the standard methods for synthesizing Trimethylsilylmethanethiol, and how do reaction conditions influence yield and purity?
this compound (C₄H₁₂SSi) is typically synthesized via nucleophilic substitution or thiolation reactions. For example, silyl chloride precursors may react with methanethiol derivatives under inert atmospheres. Reaction conditions such as temperature (e.g., 0–25°C), solvent polarity (e.g., THF or hexane), and catalysts (e.g., triethylamine) significantly affect yield and purity. Optimal conditions minimize side reactions like oxidation, which can produce disulfides. Purification via fractional distillation under reduced pressure (boiling point: ~113°C at 760 mmHg) is recommended .
Q. What spectroscopic techniques are most effective for characterizing this compound?
Key techniques include:
- ¹H/¹³C NMR : To confirm the trimethylsilyl group (δ ~0.1 ppm for Si(CH₃)₃) and thiol proton (δ ~1.3 ppm, though often broad due to exchange).
- IR Spectroscopy : S–H stretching (~2550 cm⁻¹) and Si–C bonds (~1250 cm⁻¹).
- Mass Spectrometry : Molecular ion peak at m/z 120.04 (C₄H₁₂SSi) for exact mass confirmation. Cross-referencing with CAS Common Chemistry or PubChem data ensures accuracy .
Q. How should researchers handle and store this compound to ensure stability?
Due to its low flash point (22.2°C), store in airtight containers under nitrogen at ≤4°C. Avoid exposure to moisture (to prevent hydrolysis) and oxidizing agents. Use inert gas purging during transfers and conduct work in fume hoods to mitigate inhalation risks .
Advanced Research Questions
Q. What strategies optimize this compound’s reactivity in organosilicon chemistry applications?
- Steric Shielding : The bulky trimethylsilyl group enhances regioselectivity in thiol-ene reactions by directing nucleophilic attack.
- Catalytic Activation : Use Lewis acids (e.g., BF₃·OEt₂) to stabilize transition states in substitution reactions.
- Solvent Effects : Non-polar solvents (e.g., toluene) reduce unwanted proton exchange, improving reaction specificity .
Q. How can computational chemistry methods be applied to predict reaction pathways involving this compound?
Density Functional Theory (DFT) calculations (e.g., B3LYP/6-31G*) model transition states and activation energies for thiol-based reactions. Tools like Gaussian or ORCA integrate PubChem’s molecular descriptors (e.g., bond dissociation energies) to validate pathways. Compare results with experimental kinetics (e.g., Arrhenius plots) to refine models .
Q. How should researchers address contradictions in reported reaction yields when using this compound in nucleophilic substitutions?
- Parameter Screening : Systematically vary temperature, solvent, and stoichiometry using Design of Experiments (DoE) to identify critical factors.
- Analytical Validation : Employ GC-MS or HPLC to quantify byproducts (e.g., disulfides) that may skew yield calculations.
- Meta-Analysis : Cross-reference data from Reaxys or CAS databases to identify trends in substrate compatibility .
Q. What role does this compound play in synthesizing silicon-containing polymers, and how can polymerization be controlled?
It acts as a chain-transfer agent in radical polymerizations, regulating molecular weight. Control via:
- Initiator Ratios : Adjust azobisisobutyronitrile (AIBN) concentration to balance initiation and chain transfer.
- Monomer Feed : Gradual addition of monomers (e.g., styrene) minimizes premature termination. GPC and MALDI-TOF confirm polymer architecture and end-group fidelity .
Q. Methodological and Safety Considerations
Q. How can researchers mitigate air-sensitive challenges during this compound experiments?
- Use Schlenk lines or gloveboxes for transfers.
- Pre-dry solvents (e.g., molecular sieves) and reagents.
- Monitor oxygen levels with sensors during exothermic reactions .
Q. What statistical approaches are recommended for analyzing kinetic data in this compound reactions?
- Non-linear Regression : Fit time-concentration data to rate laws (e.g., pseudo-first-order) using software like OriginLab.
- Error Propagation : Account for instrument precision (±0.1°C in thermocouples) in Arrhenius calculations .
Q. How do isotopic labeling studies (e.g., deuterated analogs) clarify this compound’s mechanistic role?
Properties
IUPAC Name |
trimethylsilylmethanethiol | |
---|---|---|
Source | PubChem | |
URL | https://pubchem.ncbi.nlm.nih.gov | |
Description | Data deposited in or computed by PubChem | |
InChI |
InChI=1S/C4H12SSi/c1-6(2,3)4-5/h5H,4H2,1-3H3 | |
Source | PubChem | |
URL | https://pubchem.ncbi.nlm.nih.gov | |
Description | Data deposited in or computed by PubChem | |
InChI Key |
IIYTXTQVIDHSSL-UHFFFAOYSA-N | |
Source | PubChem | |
URL | https://pubchem.ncbi.nlm.nih.gov | |
Description | Data deposited in or computed by PubChem | |
Canonical SMILES |
C[Si](C)(C)CS | |
Source | PubChem | |
URL | https://pubchem.ncbi.nlm.nih.gov | |
Description | Data deposited in or computed by PubChem | |
Molecular Formula |
C4H12SSi | |
Source | PubChem | |
URL | https://pubchem.ncbi.nlm.nih.gov | |
Description | Data deposited in or computed by PubChem | |
DSSTOX Substance ID |
DTXSID70171175 | |
Record name | Methanethiol, trimethylsilyl- | |
Source | EPA DSSTox | |
URL | https://comptox.epa.gov/dashboard/DTXSID70171175 | |
Description | DSSTox provides a high quality public chemistry resource for supporting improved predictive toxicology. | |
Molecular Weight |
120.29 g/mol | |
Source | PubChem | |
URL | https://pubchem.ncbi.nlm.nih.gov | |
Description | Data deposited in or computed by PubChem | |
CAS No. |
18165-76-9 | |
Record name | Methanethiol, trimethylsilyl- | |
Source | ChemIDplus | |
URL | https://pubchem.ncbi.nlm.nih.gov/substance/?source=chemidplus&sourceid=0018165769 | |
Description | ChemIDplus is a free, web search system that provides access to the structure and nomenclature authority files used for the identification of chemical substances cited in National Library of Medicine (NLM) databases, including the TOXNET system. | |
Record name | Methanethiol, trimethylsilyl- | |
Source | EPA DSSTox | |
URL | https://comptox.epa.gov/dashboard/DTXSID70171175 | |
Description | DSSTox provides a high quality public chemistry resource for supporting improved predictive toxicology. | |
Record name | (trimethylsilyl)methanethiol | |
Source | European Chemicals Agency (ECHA) | |
URL | https://echa.europa.eu/information-on-chemicals | |
Description | The European Chemicals Agency (ECHA) is an agency of the European Union which is the driving force among regulatory authorities in implementing the EU's groundbreaking chemicals legislation for the benefit of human health and the environment as well as for innovation and competitiveness. | |
Explanation | Use of the information, documents and data from the ECHA website is subject to the terms and conditions of this Legal Notice, and subject to other binding limitations provided for under applicable law, the information, documents and data made available on the ECHA website may be reproduced, distributed and/or used, totally or in part, for non-commercial purposes provided that ECHA is acknowledged as the source: "Source: European Chemicals Agency, http://echa.europa.eu/". Such acknowledgement must be included in each copy of the material. ECHA permits and encourages organisations and individuals to create links to the ECHA website under the following cumulative conditions: Links can only be made to webpages that provide a link to the Legal Notice page. | |
Retrosynthesis Analysis
AI-Powered Synthesis Planning: Our tool employs the Template_relevance Pistachio, Template_relevance Bkms_metabolic, Template_relevance Pistachio_ringbreaker, Template_relevance Reaxys, Template_relevance Reaxys_biocatalysis model, leveraging a vast database of chemical reactions to predict feasible synthetic routes.
One-Step Synthesis Focus: Specifically designed for one-step synthesis, it provides concise and direct routes for your target compounds, streamlining the synthesis process.
Accurate Predictions: Utilizing the extensive PISTACHIO, BKMS_METABOLIC, PISTACHIO_RINGBREAKER, REAXYS, REAXYS_BIOCATALYSIS database, our tool offers high-accuracy predictions, reflecting the latest in chemical research and data.
Strategy Settings
Precursor scoring | Relevance Heuristic |
---|---|
Min. plausibility | 0.01 |
Model | Template_relevance |
Template Set | Pistachio/Bkms_metabolic/Pistachio_ringbreaker/Reaxys/Reaxys_biocatalysis |
Top-N result to add to graph | 6 |
Feasible Synthetic Routes
Disclaimer and Information on In-Vitro Research Products
Please be aware that all articles and product information presented on BenchChem are intended solely for informational purposes. The products available for purchase on BenchChem are specifically designed for in-vitro studies, which are conducted outside of living organisms. In-vitro studies, derived from the Latin term "in glass," involve experiments performed in controlled laboratory settings using cells or tissues. It is important to note that these products are not categorized as medicines or drugs, and they have not received approval from the FDA for the prevention, treatment, or cure of any medical condition, ailment, or disease. We must emphasize that any form of bodily introduction of these products into humans or animals is strictly prohibited by law. It is essential to adhere to these guidelines to ensure compliance with legal and ethical standards in research and experimentation.