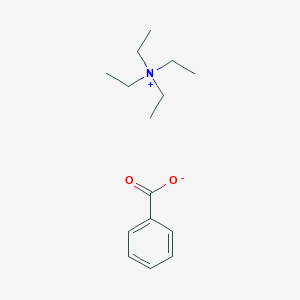
Tetraethylammonium benzoate
Overview
Description
Tetraethylammonium benzoate (TEAB, CAS 16909-22-1) is a quaternary ammonium salt composed of a tetraethylammonium cation and a benzoate anion. It is widely utilized as an initiator in the anionic ring-opening polymerization (AROP) of alkyl malolactonates to synthesize biocompatible poly(malic acid) (PMLA) derivatives for biomedical applications, such as drug delivery systems . TEAB’s commercial availability and efficiency in producing well-defined polymer architectures (e.g., hydrophobic poly(benzyl malate) or amphiphilic block copolymers) make it a cornerstone in polymer chemistry . Structural characterization of TEAB-initiated polymers is typically performed via FTIR and NMR spectroscopy, confirming high conversion rates (e.g., 100% β-lactone band disappearance in FTIR at 1850 cm⁻¹) .
Preparation Methods
Synthetic Routes and Reaction Conditions: Tetraethylammonium benzoate can be synthesized through the reaction of tetraethylammonium hydroxide with benzoic acid. The reaction typically occurs in an aqueous medium, where tetraethylammonium hydroxide neutralizes benzoic acid to form the desired salt.
Industrial Production Methods: Industrial production of this compound follows a similar synthetic route but on a larger scale. The reaction conditions are optimized to ensure high yield and purity. The product is then purified through crystallization or other suitable methods .
Chemical Reactions Analysis
Types of Reactions: Tetraethylammonium benzoate undergoes various chemical reactions, including:
Substitution Reactions: It can participate in nucleophilic substitution reactions due to the presence of the benzoate anion.
Catalytic Reactions: It acts as a bifunctional organocatalyst in reactions such as cyanosilylation of carbonyl compounds.
Common Reagents and Conditions:
Nucleophilic Substitution: Common reagents include alkyl halides and conditions typically involve mild temperatures.
Catalytic Reactions: Reactions are often carried out under solvent-free conditions at room temperature with very low catalyst loading.
Major Products:
Substitution Reactions: The major products depend on the specific nucleophile used.
Catalytic Reactions: Products include cyanosilylated carbonyl compounds and other functionalized organic molecules.
Scientific Research Applications
Chemical Properties and Structure
Tetraethylammonium benzoate (C₁₅H₂₅NO₂) consists of a tetraethylammonium cation and a benzoate anion. Its unique structure allows it to function effectively as a phase-transfer catalyst, facilitating reactions between immiscible phases in organic chemistry. This capability is crucial for enhancing reaction rates and yields in various synthetic processes.
Organic Synthesis
Phase-Transfer Catalyst
this compound is primarily recognized for its role as a phase-transfer catalyst. It enables the transfer of reactants between organic and aqueous phases, which is essential in many organic reactions. For instance, it has been effectively used in the synthesis of esters and other derivatives where reactants are in different phases.
Catalytic Efficiency
Research has demonstrated that this compound can activate substrates through Lewis acid-base interactions due to its structural characteristics. This property enhances its effectiveness in catalyzing reactions under mild conditions, making it a valuable tool in synthetic organic chemistry.
Biological Applications
Modulation of Ion Channels
this compound has shown potential in biological systems, particularly regarding its interaction with ion channels. Studies indicate that it may modulate the activity of potassium channels, which are critical for various physiological processes. This modulation can have implications for developing pharmacological agents targeting ion channel-related disorders .
Pharmacological Research
While tetraethylammonium itself has been used as a research agent to block selective potassium channels, its benzoate form is being investigated for similar applications. The compound's ability to block autonomic ganglia and calcium-activated potassium channels suggests potential therapeutic avenues, although specific clinical applications remain under exploration .
Electrochemical Applications
This compound has been utilized in electrochemical analyses due to its conductive properties. It serves as an electrolyte in various electrochemical cells, enhancing the efficiency of reactions involved in energy storage and conversion processes .
Table 1: Summary of Applications
Application Area | Description | Key Findings |
---|---|---|
Organic Synthesis | Acts as a phase-transfer catalyst | Enhances reaction rates and yields under mild conditions |
Biological Research | Modulates ion channel activity | Potential therapeutic applications being explored |
Electrochemical Analysis | Serves as an electrolyte in electrochemical cells | Improves efficiency in energy-related reactions |
Case Study: Catalytic Activity
In a study examining the catalytic efficiency of this compound in cyanosilylation reactions, it was found to catalyze the reaction of carbonyl compounds effectively at low catalyst concentrations (0.15 mol%) under mild conditions. This demonstrates its utility as a bifunctional catalyst capable of facilitating complex organic transformations efficiently .
Mechanism of Action
The mechanism of action of tetraethylammonium benzoate involves its ability to block autonomic ganglia, calcium- and voltage-activated potassium channels, and nicotinic acetylcholine receptors. This blocking action prevents the transmission of certain neural signals, leading to effects such as vasodilation . The benzoate anion also plays a role in activating reaction components as Lewis basic and Lewis acidic centers .
Comparison with Similar Compounds
Tetraethylammonium benzoate is part of a broader family of tetraethylammonium carboxylates. Key structural analogs and their comparative properties are outlined below:
Structural Analogs with Modified Benzoate Anions
Tetraethylammonium 2-(Carbamoyl)benzoate (TEACB)
- Structure : Features a carbamoyl (-CONH₂) group at the ortho position of the benzoate anion.
- Applications: A bifunctional organocatalyst for green synthesis of 4H-pyrans and Hantzsch dihydropyridines under solvent-free ball-milling conditions .
- Performance : Achieves high yields (85–95%) in short reaction times (20–40 min) due to synergistic hydrogen-bonding and electrostatic interactions .
- Advantages : Transition-metal-free, low catalyst loading (2 mol%), and cost-effective .
Tetraethylammonium 2-(N-Hydroxycarbamoyl)benzoate (TEACB-OH)
- Structure : Incorporates an N-hydroxycarbamoyl (-CONHOH) group on the benzoate anion.
- Applications: Efficient catalyst for cyanosilylation of carbonyl compounds (e.g., 95% yield in 10 min at 25°C) .
- Performance: High turnover number (TON = 950) and recyclability without loss of activity .
- Mechanism : Bifunctional activation via hydrogen bonding (N–H···O) and nucleophilic activation by the carboxylate group .
Tetraethylammonium 4-Hydroxybenzoate Monohydrate
- Structure: Contains a hydroxyl group at the para position of the benzoate anion, crystallizing as a monohydrate .
- Physical Properties : Forms a layered structure stabilized by O–H···O hydrogen bonds, influencing solubility and thermal stability .
Cation-Modified Analogs
Tetramethylammonium Benzoate (TMAB, CAS 25255-90-7)
- Structure : Smaller tetramethylammonium cation paired with benzoate.
- Applications : Less commonly used in polymerization but explored in organic synthesis.
- Comparison : Reduced steric bulk compared to TEAB may enhance solubility in polar solvents but lowers thermal stability .
Tetraheptylammonium Bromide
- Structure : Larger tetraheptylammonium cation with bromide counterion.
- Applications : Ionic liquid or phase-transfer catalyst; unrelated to carboxylate chemistry but highlights cation size effects on solubility and reactivity .
Comparative Data Table
Key Findings and Insights
Bifunctionality : TEACB and TEACB-OH outperform TEAB in organic catalysis due to dual activation modes (acid-base or hydrogen-bonding interactions) .
Cation Effects : Larger cations (e.g., tetraethyl vs. tetramethyl) enhance thermal stability but may reduce solubility in aqueous systems .
Green Chemistry : TEACB derivatives enable transition-metal-free, solvent-free syntheses, aligning with sustainable chemistry principles .
Polymerization : TEAB remains unmatched in PMLA synthesis due to its commercial availability and precise control over polymer architecture .
Biological Activity
Tetraethylammonium benzoate (TEAB) is a quaternary ammonium salt that has garnered attention for its biological activity, particularly in modulating ion channels and serving as a phase-transfer catalyst. This article delves into the compound's biological mechanisms, pharmacological properties, and applications in research.
Chemical Structure and Properties
This compound consists of a tetraethylammonium cation and a benzoate anion, represented by the chemical formula . Its quaternary ammonium structure imparts unique properties that facilitate its interaction with biological systems and organic reactions.
TEAB primarily targets several key biological components:
- Autonomic Ganglia : TEAB acts as a blocker, influencing neurotransmission by inhibiting signals in autonomic ganglia.
- Calcium- and Voltage-Activated Potassium Channels : It modulates the activity of these channels, which are crucial for various cellular processes.
- Nicotinic Acetylcholine Receptors : By blocking these receptors, TEAB can affect muscle contraction and neurotransmitter release.
These interactions lead to significant physiological effects, including vasodilation due to the inhibition of sympathetic nervous system activity .
Biochemical Pathways
The biochemical pathways affected by TEAB are still under investigation. However, its blocking action on ion channels can influence:
- Vasodilation : Resulting from reduced sympathetic tone.
- Cellular Signaling : Modulation of ion channel activity alters intracellular calcium levels, impacting various signaling pathways.
Cellular Effects
TEAB has been shown to inhibit TRPM7 channel currents in human T lymphocytes, indicating its potential role in immune modulation. This inhibition can affect calcium homeostasis and cellular responses to stimuli.
Case Studies
- Vasodilation Studies : Research has demonstrated that TEAB can induce vasodilation in animal models by blocking autonomic ganglia. This effect has implications for potential therapeutic uses in managing conditions like hypertension.
- Ion Channel Modulation : Studies indicate that TEAB effectively blocks potassium channels involved in cardiac action potentials, suggesting applications in arrhythmia management .
Applications in Research
TEAB is utilized in various scientific applications:
- Catalysis : It serves as a phase-transfer catalyst, facilitating reactions between immiscible phases in organic synthesis. Its ability to activate substrates through Lewis acid-base interactions enhances reaction efficiency.
- Pharmacological Research : Due to its ion channel blocking properties, TEAB is studied for potential therapeutic applications in cardiovascular diseases and other conditions involving dysregulated ion channel activity .
Comparative Analysis with Other Compounds
Compound Name | Structure Type | Unique Features |
---|---|---|
Tetraethylammonium chloride | Quaternary Ammonium | Commonly used to block potassium channels |
Tetraethylammonium bromide | Quaternary Ammonium | Similar uses as a pharmacological agent |
Benzyltriethylammonium chloride | Quaternary Ammonium | Often used as a phase-transfer catalyst |
Tetra-n-butylammonium bromide | Quaternary Ammonium | Known for effectiveness in organic synthesis |
Q & A
Basic Research Questions
Q. What are the primary research applications of tetraethylammonium benzoate in polymer chemistry?
TEAB is widely used as an initiator in the anionic ring-opening polymerization (ROP) of α,α,β-trisubstituted β-lactones (e.g., diMeMLABe) to synthesize biodegradable polyesters. Key methodological steps include:
- Bulk polymerization at 37–40°C for 3–7 days .
- Targeting degrees of polymerization (DP) up to 1000, yielding molar masses of 73,000–170,000 g/mol (determined by SEC in THF) .
- Achieving low dispersity (Đ ≈ 1.2–2.2) through rigorous monomer and initiator purification .
Q. How does TEAB influence polymerization kinetics compared to other initiators?
TEAB accelerates polymerization rates by 4–6× compared to tetraethylammonium (TEA) systems. This is attributed to:
- Efficient O-alkyl cleavage initiation without chain-transfer side reactions under mild conditions (40–60°C) .
- Faster propagation rates observed in malolactonate ROP, with near-quantitative conversions achieved in 3–5 days .
- Kinetic monitoring via SEC analysis and ¹H-NMR to track molar mass growth and detect terminal fumarate byproducts .
Advanced Research Questions
Q. What strategies mitigate chain-transfer reactions during TEAB-initiated polymerizations?
Chain transfer to monomer can be minimized by:
- Ultra-high purity monomers : Removal of protic impurities (e.g., water, residual acids) reduces side reactions, enabling molar masses up to 170,000 g/mol .
- Acid quenching : Adding a drop of acid post-polymerization terminates active chain ends, preventing backbiting .
- Inert atmosphere : Glovebox techniques under nitrogen/argon suppress moisture-induced transfer .
Q. How can polymer dispersity (Đ) be systematically optimized in TEAB-mediated ROP?
Dispersity control (Đ = 1.2–1.5) requires:
- Monomer-to-initiator ratios : Targeting DP 1000 with [M]/[I] = 1000:1 reduces termination events .
- Temperature modulation : Lower temperatures (40°C) slow propagation, narrowing Đ .
- Slow monomer addition : Semi-batch protocols improve uniformity in block copolymer synthesis .
Q. What analytical methods validate TEAB’s role in polymer microstructure?
- SEC with PS standards : Measures molar mass (Mn) and Đ in THF .
- ¹H-NMR : Identifies terminal benzoate groups (δ 7.5–8.0 ppm) and quantifies conversion .
- MALDI-TOF MS : Confirms chain-end fidelity and absence of cyclic oligomers .
Q. Safety and Methodological Considerations
Q. What safety protocols are critical when handling TEAB?
TEAB’s neurotoxic potential necessitates:
- Glovebox use : For all syntheses to avoid inhalation/contact .
- Neutralization protocols : Quench waste with dilute acetic acid before disposal .
- Exposure limits : Adhere to occupational limits (<0.1 mg/m³) per GHS Category 3 guidelines .
Q. How does monomer purity impact TEAB-initiated ROP outcomes?
Impurities (e.g., residual solvents, moisture) cause:
- Reduced molar masses : SEC data show Mn drops to <60,000 g/mol with 95% pure monomers .
- Broadened dispersity : Đ increases to >2.0 due to erratic propagation/termination .
Q. Emerging Applications
Q. Can TEAB enable block copolymer synthesis from β-lactones?
Yes, TEAB facilitates sequential ROP of α,α,β-trisubstituted lactones to form:
- Diblock copolymers : Using purified MLABe and diMeMLABe with Mn ≈ 120,000 g/mol .
- Statistical copolymers : Achieved by co-polymerizing lactones with varying alkyl substituents .
Q. What mechanistic insights explain TEAB’s efficiency in ROP?
Studies propose:
- Bifunctional activation : The benzoate anion stabilizes transition states via carboxylate coordination .
- Suppressed backbiting : Bulky tetraethylammonium cations sterically hinder intramolecular esterification .
Q. Data Contradictions and Resolution
Q. Why do reported molar masses for TEAB-synthesized polymers vary (73,000–170,000 g/mol)?
Discrepancies arise from:
Properties
IUPAC Name |
tetraethylazanium;benzoate | |
---|---|---|
Source | PubChem | |
URL | https://pubchem.ncbi.nlm.nih.gov | |
Description | Data deposited in or computed by PubChem | |
InChI |
InChI=1S/C8H20N.C7H6O2/c1-5-9(6-2,7-3)8-4;8-7(9)6-4-2-1-3-5-6/h5-8H2,1-4H3;1-5H,(H,8,9)/q+1;/p-1 | |
Source | PubChem | |
URL | https://pubchem.ncbi.nlm.nih.gov | |
Description | Data deposited in or computed by PubChem | |
InChI Key |
CIFIGXMZHITUAZ-UHFFFAOYSA-M | |
Source | PubChem | |
URL | https://pubchem.ncbi.nlm.nih.gov | |
Description | Data deposited in or computed by PubChem | |
Canonical SMILES |
CC[N+](CC)(CC)CC.C1=CC=C(C=C1)C(=O)[O-] | |
Source | PubChem | |
URL | https://pubchem.ncbi.nlm.nih.gov | |
Description | Data deposited in or computed by PubChem | |
Molecular Formula |
C15H25NO2 | |
Source | PubChem | |
URL | https://pubchem.ncbi.nlm.nih.gov | |
Description | Data deposited in or computed by PubChem | |
DSSTOX Substance ID |
DTXSID3066132 | |
Record name | Tetraethylammonium benzoate | |
Source | EPA DSSTox | |
URL | https://comptox.epa.gov/dashboard/DTXSID3066132 | |
Description | DSSTox provides a high quality public chemistry resource for supporting improved predictive toxicology. | |
Molecular Weight |
251.36 g/mol | |
Source | PubChem | |
URL | https://pubchem.ncbi.nlm.nih.gov | |
Description | Data deposited in or computed by PubChem | |
CAS No. |
16909-22-1 | |
Record name | Tetraethylammonium benzoate | |
Source | CAS Common Chemistry | |
URL | https://commonchemistry.cas.org/detail?cas_rn=16909-22-1 | |
Description | CAS Common Chemistry is an open community resource for accessing chemical information. Nearly 500,000 chemical substances from CAS REGISTRY cover areas of community interest, including common and frequently regulated chemicals, and those relevant to high school and undergraduate chemistry classes. This chemical information, curated by our expert scientists, is provided in alignment with our mission as a division of the American Chemical Society. | |
Explanation | The data from CAS Common Chemistry is provided under a CC-BY-NC 4.0 license, unless otherwise stated. | |
Record name | Ethanaminium, N,N,N-triethyl-, benzoate (1:1) | |
Source | ChemIDplus | |
URL | https://pubchem.ncbi.nlm.nih.gov/substance/?source=chemidplus&sourceid=0016909221 | |
Description | ChemIDplus is a free, web search system that provides access to the structure and nomenclature authority files used for the identification of chemical substances cited in National Library of Medicine (NLM) databases, including the TOXNET system. | |
Record name | Ethanaminium, N,N,N-triethyl-, benzoate (1:1) | |
Source | EPA Chemicals under the TSCA | |
URL | https://www.epa.gov/chemicals-under-tsca | |
Description | EPA Chemicals under the Toxic Substances Control Act (TSCA) collection contains information on chemicals and their regulations under TSCA, including non-confidential content from the TSCA Chemical Substance Inventory and Chemical Data Reporting. | |
Record name | Tetraethylammonium benzoate | |
Source | EPA DSSTox | |
URL | https://comptox.epa.gov/dashboard/DTXSID3066132 | |
Description | DSSTox provides a high quality public chemistry resource for supporting improved predictive toxicology. | |
Record name | Tetraethylammonium benzoate | |
Source | European Chemicals Agency (ECHA) | |
URL | https://echa.europa.eu/substance-information/-/substanceinfo/100.037.219 | |
Description | The European Chemicals Agency (ECHA) is an agency of the European Union which is the driving force among regulatory authorities in implementing the EU's groundbreaking chemicals legislation for the benefit of human health and the environment as well as for innovation and competitiveness. | |
Explanation | Use of the information, documents and data from the ECHA website is subject to the terms and conditions of this Legal Notice, and subject to other binding limitations provided for under applicable law, the information, documents and data made available on the ECHA website may be reproduced, distributed and/or used, totally or in part, for non-commercial purposes provided that ECHA is acknowledged as the source: "Source: European Chemicals Agency, http://echa.europa.eu/". Such acknowledgement must be included in each copy of the material. ECHA permits and encourages organisations and individuals to create links to the ECHA website under the following cumulative conditions: Links can only be made to webpages that provide a link to the Legal Notice page. | |
Retrosynthesis Analysis
AI-Powered Synthesis Planning: Our tool employs the Template_relevance Pistachio, Template_relevance Bkms_metabolic, Template_relevance Pistachio_ringbreaker, Template_relevance Reaxys, Template_relevance Reaxys_biocatalysis model, leveraging a vast database of chemical reactions to predict feasible synthetic routes.
One-Step Synthesis Focus: Specifically designed for one-step synthesis, it provides concise and direct routes for your target compounds, streamlining the synthesis process.
Accurate Predictions: Utilizing the extensive PISTACHIO, BKMS_METABOLIC, PISTACHIO_RINGBREAKER, REAXYS, REAXYS_BIOCATALYSIS database, our tool offers high-accuracy predictions, reflecting the latest in chemical research and data.
Strategy Settings
Precursor scoring | Relevance Heuristic |
---|---|
Min. plausibility | 0.01 |
Model | Template_relevance |
Template Set | Pistachio/Bkms_metabolic/Pistachio_ringbreaker/Reaxys/Reaxys_biocatalysis |
Top-N result to add to graph | 6 |
Feasible Synthetic Routes
Disclaimer and Information on In-Vitro Research Products
Please be aware that all articles and product information presented on BenchChem are intended solely for informational purposes. The products available for purchase on BenchChem are specifically designed for in-vitro studies, which are conducted outside of living organisms. In-vitro studies, derived from the Latin term "in glass," involve experiments performed in controlled laboratory settings using cells or tissues. It is important to note that these products are not categorized as medicines or drugs, and they have not received approval from the FDA for the prevention, treatment, or cure of any medical condition, ailment, or disease. We must emphasize that any form of bodily introduction of these products into humans or animals is strictly prohibited by law. It is essential to adhere to these guidelines to ensure compliance with legal and ethical standards in research and experimentation.