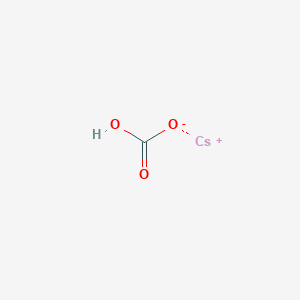
Cesium bicarbonate
Overview
Description
Cesium bicarbonate (CsHCO₃), also known as cesium hydrogen carbonate, is an alkali metal bicarbonate with the linear formula CsH₂CO₃ . This compound is synthesized via reactions involving cesium carbonate (Cs₂CO₃) or cesium hydroxide (CsOH) with carbon dioxide.
Preparation Methods
Synthetic Routes and Reaction Conditions: Cesium bicarbonate can be synthesized through the reaction of cesium carbonate with carbon dioxide and water:
Cs2CO3+CO2+H2O→2CsHCO3
Alternatively, it can be prepared by passing carbon dioxide through a concentrated solution of cesium hydroxide or cesium carbonate .
Industrial Production Methods: In industrial settings, this compound is typically produced by bubbling carbon dioxide through a solution of cesium carbonate. The reaction is carried out under controlled conditions to ensure the complete conversion of cesium carbonate to this compound .
Chemical Reactions Analysis
Types of Reactions: Cesium bicarbonate undergoes various chemical reactions, including:
Decomposition: Upon heating, this compound decomposes to form cesium carbonate, water, and carbon dioxide.
2CsHCO3→Cs2CO3+H2O+CO2
Acid-Base Reactions: this compound reacts with acids to form cesium salts, water, and carbon dioxide.
CsHCO3+HCl→CsCl+H2O+CO2
Common Reagents and Conditions:
Reagents: Common reagents include acids like hydrochloric acid and sulfuric acid.
Conditions: Reactions are typically carried out at room temperature or slightly elevated temperatures.
Major Products:
Cesium Salts: The reaction with acids produces various cesium salts.
Cesium Carbonate: Decomposition of this compound yields cesium carbonate
Scientific Research Applications
Applications in Organic Synthesis
Cesium bicarbonate has been increasingly recognized for its role as a catalyst in organic transformations. Below are some notable applications:
Regioselective Alkylation
A significant application of this compound is its use in the regioselective alkylation of 2,4-dihydroxybenzyaldehydes and 2,4-dihydroxyacetophenones. This reaction yields 4-alkylated products with high regioselectivity and excellent yields (up to 95%) when conducted in acetonitrile at elevated temperatures .
Table 1: Summary of Alkylation Results Using this compound
Substrate | Alkylating Agent | Yield (%) | Solvent | Temperature (°C) |
---|---|---|---|---|
2,4-Dihydroxybenzyaldehyde | 1,2-Dibromoethane | 95 | Acetonitrile | 80 |
2,4-Dihydroxyacetophenone | Various | Up to 95 | Acetonitrile | 80 |
This method demonstrates the effectiveness of this compound compared to other bases, as it minimizes side reactions and enhances product yield due to its softer Lewis acidity .
Buffering Agent in Analytical Chemistry
In analytical chemistry, this compound serves as a buffering agent. Its ability to maintain pH stability makes it valuable in various analytical procedures where precise pH control is crucial .
Catalyst for Amidation Reactions
This compound has been employed as a catalyst for the direct amidation of unactivated esters with amino alcohols. This reaction occurs without the need for transition-metal catalysts, showcasing this compound's utility in facilitating amide bond formation under mild conditions .
Case Study 1: Alkylation of Hydroxy Compounds
In a study published in Organic Letters, researchers demonstrated that using this compound significantly improved the regioselectivity of alkylation reactions involving hydroxy compounds. The study highlighted how cesium ions enhance solubility and reduce coordination interactions that can lead to undesired byproducts .
Case Study 2: Buffering Applications
Research conducted on the use of this compound as a buffering agent illustrated its effectiveness in maintaining stable pH levels during complex biochemical assays. This property is particularly beneficial in experiments involving sensitive biological molecules that require stringent pH conditions for optimal activity .
Mechanism of Action
The mechanism by which cesium bicarbonate exerts its effects is primarily through the release of cesium ions (Cs⁺) in aqueous solutions. These ions can interact with various molecular targets, including enzymes and ion channels, altering their activity and function. The unique properties of cesium ions, such as their large ionic radius and high reactivity, contribute to the compound’s effectiveness in various applications .
Comparison with Similar Compounds
Comparison with Similar Alkali Metal Bicarbonates
Cesium bicarbonate belongs to the alkali metal bicarbonate group, which includes sodium bicarbonate (NaHCO₃), potassium bicarbonate (KHCO₃), and rubidium bicarbonate (RbHCO₃). Below is a detailed comparison based on chemical properties, stability, and applications.
Physical and Chemical Properties
*Cesium salts generally exhibit higher solubility due to the low charge density of Cs⁺ ions. Evidence suggests CsHCO₃ has improved solubility compared to NaHCO₃, making it advantageous in hyperpolarized MRI techniques, though toxicity concerns persist .
Reactivity and Stability
- This compound : Acts as a mild base in organic synthesis. In toluene, cesium carbonate (Cs₂CO₃, a related compound) behaves heterogeneously due to low solubility, but CsHCO₃ may dissolve better in polar solvents .
- Sodium Bicarbonate : Widely used as a buffering agent due to its moderate alkalinity and stability. It decomposes at lower temperatures, releasing CO₂, which is utilized in baking and fire extinguishers .
- Potassium Bicarbonate : Similar to NaHCO₃ but with higher solubility, making it preferable in agriculture (e.g., fungicides) and food preservation .
Key Research Findings
- Synthetic Utility : this compound’s high solubility enhances its efficacy in reactions requiring homogeneous basic conditions, such as palladium-catalyzed alcohol resolutions. However, granular Cs₂CO₃ (a precursor) often performs better in heterogeneous systems .
- Medical Imaging : Hyperpolarized CsHCO₃ improves lung pH mapping accuracy but is overshadowed by sodium-based alternatives due to safety concerns .
Biological Activity
Cesium bicarbonate (CsHCO₃) is a compound that has garnered attention in various scientific studies for its biological activity, particularly its effects on cellular metabolism and potential therapeutic applications. This article synthesizes findings from diverse research sources, focusing on the biological implications of this compound, including its impact on glycolytic pathways, cellular proliferation, and potential therapeutic uses.
Overview of this compound
This compound is a salt formed from cesium and bicarbonate ions. It is known for its unique ionic properties, which influence its behavior in biological systems. Cesium ions (Cs⁺) are similar in size to potassium ions (K⁺), allowing them to interact with biological processes that typically involve potassium.
Effects on Glycolysis
Recent studies have highlighted the role of this compound in modulating glycolytic pathways. A significant study examined the effects of cesium treatment on HeLa cells, a type of cervical cancer cell line. The research indicated that cesium ions inhibit key glycolytic enzymes, leading to decreased cell proliferation:
- Glycolytic Enzyme Activity : The study measured the activity of several glycolytic enzymes, including hexokinase (HK), glyceraldehyde-3-phosphate dehydrogenase (GAPDH), and pyruvate kinase (PK). Results showed that cesium treatment resulted in a significant reduction in the activity of these enzymes compared to control cells:
- HK Activity : 0.03 µmol NADPH/min/mg-protein (Cs-treated) vs. 0.03 µmol NADPH/min/mg-protein (control)
- GAPDH Activity : 0.88 µmol NADH/min/mg-protein (Cs-treated) vs. 0.32 µmol NADH/min/mg-protein (control)
- PK Activity : 0.12 µmol NADH/min/mg-protein (Cs-treated) vs. 0.10 µmol NADH/min/mg-protein (control) .
These findings suggest that this compound may suppress glycolysis through inhibition of enzyme activity, potentially offering insights into its use as an anticancer agent.
Case Study: Anticancer Properties
A case study focused on the anticancer properties of this compound involved its administration in patients with advanced cancer. The results indicated that patients experienced reductions in tumor size and improvements in metabolic parameters, possibly due to altered glycolytic activity induced by cesium treatment .
In Vitro Studies
In vitro studies have demonstrated that this compound can induce apoptosis in cancer cells through mechanisms involving oxidative stress and mitochondrial dysfunction. The compound's ability to disrupt normal cellular metabolism has been posited as a mechanism for its anticancer effects .
Comparative Analysis of Biological Effects
The following table summarizes key findings from various studies regarding the biological effects of this compound:
Study | Cell Type | Key Findings | Implications |
---|---|---|---|
HeLa Cell Study | HeLa Cells | Inhibition of glycolytic enzymes | Potential use in cancer therapy |
Anticancer Case Study | Cancer Patients | Tumor size reduction | Suggests therapeutic efficacy |
In Vitro Apoptosis Study | Various Cancer Cells | Induction of apoptosis via oxidative stress | Highlights mechanisms for anticancer activity |
The mechanisms through which this compound exerts its biological effects include:
- Ion Substitution : Cs⁺ can substitute for K⁺ in various enzymatic reactions, potentially leading to altered enzyme kinetics and metabolic pathways.
- Enzyme Inhibition : Cesium ions have been shown to inhibit key glycolytic enzymes, leading to reduced ATP production and energy deficits in rapidly proliferating cells.
- Oxidative Stress Induction : Cesium treatment has been associated with increased levels of reactive oxygen species (ROS), contributing to apoptosis in cancer cells .
Q & A
Basic Research Questions
Q. How can researchers experimentally determine the solubility of cesium bicarbonate under varying temperatures, and what methodologies ensure accuracy?
To determine solubility, researchers should use gravimetric analysis combined with temperature-controlled saturation experiments. Prepare saturated solutions of CsHCO₃ in deionized water at incremental temperatures (e.g., 25–200°C). Filter undissolved solids, dry, and weigh them to calculate solubility. High-temperature studies (>100°C) require pressurized reactors to prevent solvent evaporation. For validation, compare results with phase diagrams or literature data (e.g., solubility at 200°C in Figure 1 of ) .
Q. What protocols are recommended for synthesizing this compound in anhydrous conditions?
Synthesize CsHCO₃ by reacting cesium carbonate (Cs₂CO₃) with CO₂ in a dry dimethyl ether (DME) solvent under reflux. Maintain a 1:1 molar ratio of Cs₂CO₃ to CO₂ to avoid excess bicarbonate formation. Monitor reaction completion via pH titration or gas evolution (CO₂ release). Post-synthesis, isolate CsHCO₃ via vacuum filtration and dry under inert gas flow .
Q. How can impurities in this compound samples be quantified, and what analytical techniques are optimal?
Use inductively coupled plasma mass spectrometry (ICP-MS) to detect trace metal impurities (e.g., Na⁺, K⁺). For organic contaminants, employ gas chromatography-mass spectrometry (GC-MS). Purity validation requires X-ray diffraction (XRD) to confirm crystallinity and Fourier-transform infrared spectroscopy (FTIR) to identify bicarbonate functional groups (e.g., asymmetric C-O stretching at ~1650 cm⁻¹) .
Advanced Research Questions
Q. How does this compound influence the kinetics of CO₂ electroreduction compared to other alkali bicarbonates?
CsHCO₃ enhances CO₂ reduction reaction (CO2RR) efficiency by suppressing hydrogen evolution (HER) due to its large cation size (Cs⁺), which weakens H⁺ adsorption on electrodes. Design experiments using a three-electrode cell with CsHCO₃-based electrolytes (e.g., 0.02 M CsHCO₃ + 0.08 M KHCO₃). Measure Faradaic efficiency via gas chromatography and compare with NaHCO₃ or KHCO₃ controls. The higher pH buffering capacity of CsHCO₃ also stabilizes reaction intermediates .
Q. What mechanisms explain this compound’s role in enhancing perovskite nanocrystal stability for optoelectronic applications?
CsHCO₃ acts as a precursor in synthesizing cesium lead halide perovskites (CsPbX₃). Its decomposition during annealing releases CO₂, creating porous structures that reduce lattice strain. To test this, prepare CsPbBr₃ films using CsHCO₃ and compare photoluminescence quantum yield (PLQY) and degradation rates under UV exposure with films made from Cs₂CO₃. The bicarbonate route reduces halide vacancies, improving stability .
Q. How can this compound be optimized for non-acid elution of cesium isotopes in nuclear waste remediation?
In column chromatography, use 3M Rb₂CO₃ or NH₄HCO₃ solutions to elute Cs⁺ from resorcinol-based resins. CsHCO₃’s low Kd (partition coefficient) values (~2–7 L/kg) indicate high elution efficiency. Validate via ICP-MS by measuring Cs⁺ concentration in eluates and comparing with target values. Note that excess NH₄HCO₃ may lower Cs⁺ recovery due to competitive ion effects .
Q. Data Contradictions and Resolution
Q. Why do studies report conflicting results on this compound’s efficacy in metastasis inhibition compared to sodium bicarbonate?
While NaHCO₃ reduces tumor acidity and metastasis in breast cancer models ( ), CsHCO₃’s larger ion size may limit cellular uptake, reducing its buffering capacity. To resolve contradictions, replicate in vivo studies using CsHCO₃ oral dosing in metastatic models (e.g., PC3M prostate cancer). Measure extracellular tumor pH via ³¹P magnetic resonance spectroscopy and compare metastasis rates with NaHCO₃-treated cohorts .
Q. How can researchers address discrepancies in this compound’s solubility data across literature sources?
Discrepancies arise from variations in experimental conditions (e.g., pressure, solvent purity). Standardize measurements using IUPAC-recommended protocols, such as isothermal saturation methods in sealed reactors. Cross-validate with computational models (e.g., Pitzer equations) to predict solubility under non-ideal conditions .
Q. Methodological Best Practices
Q. What precautions are critical when handling this compound in high-temperature reactions?
- Use sealed reactors to prevent CO₂ loss, which alters reaction stoichiometry.
- Monitor pressure changes to avoid explosive decomposition (CsHCO₃ → Cs₂CO₃ + CO₂ + H₂O).
- Post-reaction, quench samples in inert atmospheres to preserve products .
Q. How should researchers design controls when studying CsHCO₃’s role in ionic transport mechanisms?
Properties
CAS No. |
15519-28-5 |
---|---|
Molecular Formula |
CH2CsO3 |
Molecular Weight |
194.930 g/mol |
IUPAC Name |
cesium;hydrogen carbonate |
InChI |
InChI=1S/CH2O3.Cs/c2-1(3)4;/h(H2,2,3,4); |
InChI Key |
UHKPOGGUWJGGID-UHFFFAOYSA-N |
SMILES |
C(=O)(O)[O-].[Cs+] |
Isomeric SMILES |
C(=O)(O)[O-].[Cs+] |
Canonical SMILES |
C(=O)(O)O.[Cs] |
Key on ui other cas no. |
15519-28-5 29703-01-3 |
Pictograms |
Irritant |
Related CAS |
29703-01-3 |
Origin of Product |
United States |
Retrosynthesis Analysis
AI-Powered Synthesis Planning: Our tool employs the Template_relevance Pistachio, Template_relevance Bkms_metabolic, Template_relevance Pistachio_ringbreaker, Template_relevance Reaxys, Template_relevance Reaxys_biocatalysis model, leveraging a vast database of chemical reactions to predict feasible synthetic routes.
One-Step Synthesis Focus: Specifically designed for one-step synthesis, it provides concise and direct routes for your target compounds, streamlining the synthesis process.
Accurate Predictions: Utilizing the extensive PISTACHIO, BKMS_METABOLIC, PISTACHIO_RINGBREAKER, REAXYS, REAXYS_BIOCATALYSIS database, our tool offers high-accuracy predictions, reflecting the latest in chemical research and data.
Strategy Settings
Precursor scoring | Relevance Heuristic |
---|---|
Min. plausibility | 0.01 |
Model | Template_relevance |
Template Set | Pistachio/Bkms_metabolic/Pistachio_ringbreaker/Reaxys/Reaxys_biocatalysis |
Top-N result to add to graph | 6 |
Feasible Synthetic Routes
Disclaimer and Information on In-Vitro Research Products
Please be aware that all articles and product information presented on BenchChem are intended solely for informational purposes. The products available for purchase on BenchChem are specifically designed for in-vitro studies, which are conducted outside of living organisms. In-vitro studies, derived from the Latin term "in glass," involve experiments performed in controlled laboratory settings using cells or tissues. It is important to note that these products are not categorized as medicines or drugs, and they have not received approval from the FDA for the prevention, treatment, or cure of any medical condition, ailment, or disease. We must emphasize that any form of bodily introduction of these products into humans or animals is strictly prohibited by law. It is essential to adhere to these guidelines to ensure compliance with legal and ethical standards in research and experimentation.