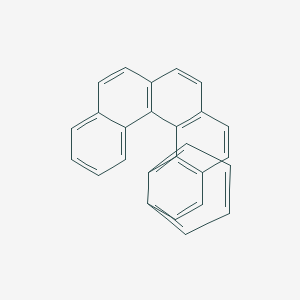
Hexahelicene
Overview
Description
Hexahelicene is a polycyclic aromatic hydrocarbon characterized by its unique helical structure. It consists of six ortho-fused benzene rings that form a non-planar, screw-shaped molecule. This helical structure imparts chirality to the molecule, making it an interesting subject of study in stereochemistry. The systematic name for this compound is phenanthro[3,4-c]phenanthrene .
Preparation Methods
Synthetic Routes and Reaction Conditions: Hexahelicene can be synthesized through several methods, with the most common being the oxidative photocyclization of stilbene-like precursors. This method involves the cyclization of a stilbene derivative under oxidative conditions, typically using iodine or oxygen as the oxidizing agent . Another common method is the Diels-Alder reaction, which involves the cycloaddition of a diene and a dienophile to form the helical structure .
Industrial Production Methods: While this compound is primarily synthesized in research laboratories, industrial production methods would likely involve scaling up the oxidative photocyclization or Diels-Alder reactions. These methods would need to be optimized for yield, purity, and cost-effectiveness to be viable on an industrial scale .
Chemical Reactions Analysis
Types of Reactions: Hexahelicene undergoes various chemical reactions, including:
Oxidation: this compound can be oxidized to form this compound quinone.
Reduction: Reduction of this compound can yield dihydrothis compound.
Substitution: Electrophilic aromatic substitution reactions can introduce various functional groups onto the this compound framework.
Common Reagents and Conditions:
Oxidation: Common oxidizing agents include iodine and oxygen.
Reduction: Reducing agents such as sodium borohydride or lithium aluminum hydride can be used.
Substitution: Reagents like bromine, chlorine, or nitrating agents can be used for electrophilic aromatic substitution.
Major Products:
Oxidation: this compound quinone.
Reduction: Dihydrothis compound.
Substitution: Various substituted hexahelicenes depending on the reagent used.
Scientific Research Applications
Hexahelicene has a wide range of applications in scientific research:
Mechanism of Action
The unique helical structure of hexahelicene allows it to interact with various molecular targets through π-π stacking interactions and other non-covalent interactions. These interactions can influence the activity of enzymes, receptors, and other biological molecules. The chirality of this compound also plays a crucial role in its mechanism of action, as the two enantiomers can have different biological activities .
Comparison with Similar Compounds
Hexahelicene is part of a larger family of helicenes, which are characterized by their helical structures. Similar compounds include:
Pentahelicene: Consists of five ortho-fused benzene rings.
Heptahelicene: Consists of seven ortho-fused benzene rings.
Octahelicene: Consists of eight ortho-fused benzene rings.
Uniqueness of this compound: this compound is unique due to its balance between structural complexity and synthetic accessibility. Its six-ring structure provides sufficient helical twist to exhibit interesting chiral properties, while still being relatively straightforward to synthesize compared to longer helicenes .
Biological Activity
Hexahelicene is a member of the helicene family, characterized by its unique helical structure formed by six ortho-fused benzene rings. This compound has garnered attention for its potential biological activities, particularly in the fields of medicinal chemistry and materials science. The following sections will delve into the biological activity of this compound, including its interactions with biological systems, cytotoxicity, and potential therapeutic applications.
Structure and Properties
This compound exhibits a chiral structure that can exist in two enantiomeric forms. Its unique geometry contributes to its electronic properties, making it an interesting candidate for various applications in organic electronics and photonics. The compound's ability to form complexes with metal ions, such as silver, has also been investigated for potential biomedical applications .
Cytotoxicity and Biological Interaction
Recent studies have explored the cytotoxic effects of this compound on various cell lines. A significant investigation reported that this compound demonstrates low cytotoxicity against HepG2 liver cancer cells, indicating it may not be a potent activator of the aryl hydrocarbon receptor (AhR) pathway . This finding suggests that while this compound may have some biological activity, its effectiveness as a cancer therapeutic agent requires further exploration.
Table 1: Cytotoxicity of this compound
Cell Line | IC50 (µM) | Mechanism of Action |
---|---|---|
HepG2 | >100 | Poor AhR pathway activator |
MCF-7 | TBD | TBD |
A549 | TBD | TBD |
Complexation with Metal Ions
The interaction of this compound with metal ions has been a focal point in understanding its potential as a drug delivery system. Studies indicate that this compound can form stable complexes with silver ions (Ag+), which may enhance its biological activity. The complexation process has been shown to be endothermic, suggesting that temperature increases can enhance the adsorption capacity of this compound for metal ions .
Case Study: Silver-Hexahelicene Complex
A recent study investigated the complexation of silver ions with this compound using various adsorbates. The results indicated that silver chloride (AgCl) presented the highest adsorption capacity when complexed with this compound. This suggests that this compound could function as a chiral molecular tweezer for silver ions, potentially leading to applications in targeted drug delivery systems for cancer treatment .
Optical Properties and Applications
This compound and its derivatives have been studied for their optical properties, particularly in circularly polarized luminescence (CPL). Research has shown that modifications to the helical structure can significantly enhance fluorescence quantum yields, making BN-hexahelicenes promising candidates for CPL emitters in materials science applications . The incorporation of boron-nitrogen (BN) units into hexahelicenes has resulted in improved luminescence properties compared to their all-carbon analogs.
Table 2: Optical Properties of Hexahelicenes
| Compound | Fluorescence Quantum Yield (φfl) | Luminescence Dissymmetry Factor (|g lum|) |
|------------------|----------------------------------|------------------------------------------|
| this compound | 0.04 | 9 × 10^-4 |
| BN-Hexahelicene | 0.21 | 1.33 × 10^-2 |
Properties
IUPAC Name |
hexahelicene | |
---|---|---|
Source | PubChem | |
URL | https://pubchem.ncbi.nlm.nih.gov | |
Description | Data deposited in or computed by PubChem | |
InChI |
InChI=1S/C26H16/c1-3-7-22-17(5-1)9-11-19-13-15-21-16-14-20-12-10-18-6-2-4-8-23(18)25(20)26(21)24(19)22/h1-16H | |
Source | PubChem | |
URL | https://pubchem.ncbi.nlm.nih.gov | |
Description | Data deposited in or computed by PubChem | |
InChI Key |
UOYPNWSDSPYOSN-UHFFFAOYSA-N | |
Source | PubChem | |
URL | https://pubchem.ncbi.nlm.nih.gov | |
Description | Data deposited in or computed by PubChem | |
Canonical SMILES |
C1=CC=C2C(=C1)C=CC3=C2C4=C(C=C3)C=CC5=C4C6=CC=CC=C6C=C5 | |
Source | PubChem | |
URL | https://pubchem.ncbi.nlm.nih.gov | |
Description | Data deposited in or computed by PubChem | |
Molecular Formula |
C26H16 | |
Source | PubChem | |
URL | https://pubchem.ncbi.nlm.nih.gov | |
Description | Data deposited in or computed by PubChem | |
DSSTOX Substance ID |
DTXSID80171964 | |
Record name | Hexahelicene | |
Source | EPA DSSTox | |
URL | https://comptox.epa.gov/dashboard/DTXSID80171964 | |
Description | DSSTox provides a high quality public chemistry resource for supporting improved predictive toxicology. | |
Molecular Weight |
328.4 g/mol | |
Source | PubChem | |
URL | https://pubchem.ncbi.nlm.nih.gov | |
Description | Data deposited in or computed by PubChem | |
CAS No. |
187-83-7 | |
Record name | [6]Helicene | |
Source | CAS Common Chemistry | |
URL | https://commonchemistry.cas.org/detail?cas_rn=187-83-7 | |
Description | CAS Common Chemistry is an open community resource for accessing chemical information. Nearly 500,000 chemical substances from CAS REGISTRY cover areas of community interest, including common and frequently regulated chemicals, and those relevant to high school and undergraduate chemistry classes. This chemical information, curated by our expert scientists, is provided in alignment with our mission as a division of the American Chemical Society. | |
Explanation | The data from CAS Common Chemistry is provided under a CC-BY-NC 4.0 license, unless otherwise stated. | |
Record name | Hexahelicene | |
Source | ChemIDplus | |
URL | https://pubchem.ncbi.nlm.nih.gov/substance/?source=chemidplus&sourceid=0000187837 | |
Description | ChemIDplus is a free, web search system that provides access to the structure and nomenclature authority files used for the identification of chemical substances cited in National Library of Medicine (NLM) databases, including the TOXNET system. | |
Record name | Hexahelicene | |
Source | DTP/NCI | |
URL | https://dtp.cancer.gov/dtpstandard/servlet/dwindex?searchtype=NSC&outputformat=html&searchlist=171474 | |
Description | The NCI Development Therapeutics Program (DTP) provides services and resources to the academic and private-sector research communities worldwide to facilitate the discovery and development of new cancer therapeutic agents. | |
Explanation | Unless otherwise indicated, all text within NCI products is free of copyright and may be reused without our permission. Credit the National Cancer Institute as the source. | |
Record name | Hexahelicene | |
Source | EPA DSSTox | |
URL | https://comptox.epa.gov/dashboard/DTXSID80171964 | |
Description | DSSTox provides a high quality public chemistry resource for supporting improved predictive toxicology. | |
Record name | Hexahelicene | |
Source | FDA Global Substance Registration System (GSRS) | |
URL | https://gsrs.ncats.nih.gov/ginas/app/beta/substances/G5UR49H4NR | |
Description | The FDA Global Substance Registration System (GSRS) enables the efficient and accurate exchange of information on what substances are in regulated products. Instead of relying on names, which vary across regulatory domains, countries, and regions, the GSRS knowledge base makes it possible for substances to be defined by standardized, scientific descriptions. | |
Explanation | Unless otherwise noted, the contents of the FDA website (www.fda.gov), both text and graphics, are not copyrighted. They are in the public domain and may be republished, reprinted and otherwise used freely by anyone without the need to obtain permission from FDA. Credit to the U.S. Food and Drug Administration as the source is appreciated but not required. | |
Synthesis routes and methods
Procedure details
Retrosynthesis Analysis
AI-Powered Synthesis Planning: Our tool employs the Template_relevance Pistachio, Template_relevance Bkms_metabolic, Template_relevance Pistachio_ringbreaker, Template_relevance Reaxys, Template_relevance Reaxys_biocatalysis model, leveraging a vast database of chemical reactions to predict feasible synthetic routes.
One-Step Synthesis Focus: Specifically designed for one-step synthesis, it provides concise and direct routes for your target compounds, streamlining the synthesis process.
Accurate Predictions: Utilizing the extensive PISTACHIO, BKMS_METABOLIC, PISTACHIO_RINGBREAKER, REAXYS, REAXYS_BIOCATALYSIS database, our tool offers high-accuracy predictions, reflecting the latest in chemical research and data.
Strategy Settings
Precursor scoring | Relevance Heuristic |
---|---|
Min. plausibility | 0.01 |
Model | Template_relevance |
Template Set | Pistachio/Bkms_metabolic/Pistachio_ringbreaker/Reaxys/Reaxys_biocatalysis |
Top-N result to add to graph | 6 |
Feasible Synthetic Routes
Disclaimer and Information on In-Vitro Research Products
Please be aware that all articles and product information presented on BenchChem are intended solely for informational purposes. The products available for purchase on BenchChem are specifically designed for in-vitro studies, which are conducted outside of living organisms. In-vitro studies, derived from the Latin term "in glass," involve experiments performed in controlled laboratory settings using cells or tissues. It is important to note that these products are not categorized as medicines or drugs, and they have not received approval from the FDA for the prevention, treatment, or cure of any medical condition, ailment, or disease. We must emphasize that any form of bodily introduction of these products into humans or animals is strictly prohibited by law. It is essential to adhere to these guidelines to ensure compliance with legal and ethical standards in research and experimentation.