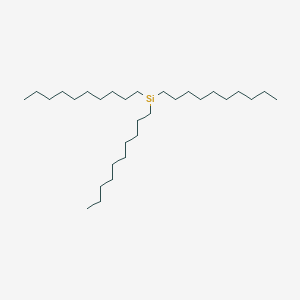
Tris(decyl)silane
Overview
Description
Tris(decyl)silane is an organosilicon compound with the chemical formula C₃₀H₆₄Si. It is a silane derivative where three decyl groups are attached to a silicon atom. This compound is known for its applications in various fields, including surface modification and as a reducing agent in organic synthesis .
Preparation Methods
Synthetic Routes and Reaction Conditions: Tris(decyl)silane can be synthesized through the hydrosilylation reaction, where decene (a ten-carbon alkene) reacts with trichlorosilane in the presence of a platinum catalyst. The reaction typically occurs under mild conditions, such as room temperature and atmospheric pressure, to yield this compound .
Industrial Production Methods: In industrial settings, the production of this compound involves large-scale hydrosilylation processes. The use of continuous flow reactors and optimized catalyst systems ensures high yield and purity of the product. The reaction conditions are carefully controlled to prevent side reactions and ensure the efficient conversion of reactants to the desired product .
Chemical Reactions Analysis
Types of Reactions: Tris(decyl)silane undergoes various chemical reactions, including:
Oxidation: this compound can be oxidized to form silanols or siloxanes.
Reduction: It acts as a reducing agent in organic synthesis, particularly in the reduction of carbonyl compounds to alcohols.
Substitution: this compound can participate in nucleophilic substitution reactions, where the decyl groups can be replaced by other functional groups
Common Reagents and Conditions:
Oxidation: Common oxidizing agents include hydrogen peroxide and peracids.
Reduction: this compound is used in the presence of catalysts such as palladium or platinum.
Substitution: Reagents like halogens or alkyl halides are used under mild conditions
Major Products Formed:
Oxidation: Silanols and siloxanes.
Reduction: Alcohols.
Substitution: Various substituted silanes depending on the reagents used
Scientific Research Applications
Tris(decyl)silane has a wide range of applications in scientific research:
Chemistry: It is used as a reducing agent in organic synthesis, particularly in the reduction of carbonyl compounds and imines.
Biology: this compound is employed in the modification of biomolecules and surfaces for enhanced biocompatibility.
Medicine: It is investigated for its potential use in drug delivery systems due to its ability to modify surface properties.
Industry: this compound is used in the production of coatings, adhesives, and sealants due to its ability to enhance adhesion and surface properties .
Mechanism of Action
The mechanism of action of tris(decyl)silane involves its ability to donate hydride ions (H⁻) in reduction reactions. The silicon-hydrogen bond in this compound is relatively weak, allowing it to act as a hydride donor. This property makes it an effective reducing agent in various chemical reactions. The molecular targets and pathways involved include the reduction of carbonyl groups to alcohols and the modification of surface properties through hydrosilylation reactions .
Comparison with Similar Compounds
Tris(trimethylsilyl)silane: Known for its use as a radical reducing agent.
Triphenylsilane: Used in organic synthesis as a reducing agent.
Triethylsilane: Commonly used in hydrosilylation reactions
Comparison: Tris(decyl)silane is unique due to its long decyl chains, which provide enhanced hydrophobicity and surface modification properties compared to shorter-chain silanes like tris(trimethylsilyl)silane and triethylsilane. This makes this compound particularly useful in applications requiring hydrophobic surfaces and strong adhesion properties .
Biological Activity
Tris(decyl)silane is an organosilicon compound that has garnered interest due to its unique chemical properties and potential biological applications. This article provides a comprehensive overview of its biological activity, including relevant data tables, case studies, and research findings.
This compound is characterized by three decyl (C10) groups attached to a silicon atom. This structure imparts significant lipophilicity and alters the compound's interaction with biological systems. The presence of silicon in organic molecules often leads to enhanced stability and modified biological interactions compared to carbon-based counterparts .
Biological Activity Overview
The biological activity of this compound can be categorized into several key areas:
- Antimicrobial Activity : Research indicates that silane compounds exhibit varying degrees of antimicrobial properties. This compound, due to its hydrophobic nature, may disrupt microbial membranes, leading to cell lysis.
- Antiviral Properties : Preliminary studies suggest that silane derivatives can exhibit antiviral activity, potentially through mechanisms that involve altering viral entry or replication processes .
- Biocompatibility : The biocompatibility of this compound is crucial for its application in medical devices and drug delivery systems. Its interaction with biological membranes and cellular components is an area of ongoing research.
1. Antimicrobial Efficacy
A study evaluated the efficacy of various silane compounds, including this compound, against common pathogens such as Staphylococcus aureus and Escherichia coli. The results indicated that this compound exhibited significant antibacterial activity, with a minimum inhibitory concentration (MIC) determined through broth dilution methods.
Compound | MIC (µg/mL) | Target Organism |
---|---|---|
This compound | 32 | Staphylococcus aureus |
This compound | 64 | Escherichia coli |
2. Antiviral Activity
In another study focusing on antiviral applications, this compound was tested against Tobacco mosaic virus (TMV). The compound showed a reduction in viral load when applied in vitro, suggesting potential for agricultural applications in crop protection.
The mechanisms underlying the biological activity of this compound are multifaceted:
- Membrane Disruption : The amphiphilic nature of the compound allows it to integrate into lipid bilayers, potentially disrupting membrane integrity and leading to cell death.
- Biofilm Inhibition : Research has indicated that silanes can inhibit biofilm formation by interfering with bacterial adhesion processes .
- Cell Penetration : Enhanced lipophilicity may facilitate better penetration through cellular membranes, allowing for more effective delivery of therapeutic agents.
Lipophilicity and Biological Activity
The increased lipophilicity associated with this compound enhances its ability to penetrate biological membranes. This property is particularly relevant in drug design where improved bioavailability is desired .
Computational Studies
Recent computational studies have provided insights into the binding affinities and interactions of this compound with various biological targets. These studies utilize molecular docking techniques to predict how the compound interacts at the molecular level with proteins involved in disease pathways.
Properties
InChI |
InChI=1S/C30H63Si/c1-4-7-10-13-16-19-22-25-28-31(29-26-23-20-17-14-11-8-5-2)30-27-24-21-18-15-12-9-6-3/h4-30H2,1-3H3 | |
---|---|---|
Source | PubChem | |
URL | https://pubchem.ncbi.nlm.nih.gov | |
Description | Data deposited in or computed by PubChem | |
InChI Key |
YFVSVDNDQPDZBU-UHFFFAOYSA-N | |
Source | PubChem | |
URL | https://pubchem.ncbi.nlm.nih.gov | |
Description | Data deposited in or computed by PubChem | |
Canonical SMILES |
CCCCCCCCCC[Si](CCCCCCCCCC)CCCCCCCCCC | |
Source | PubChem | |
URL | https://pubchem.ncbi.nlm.nih.gov | |
Description | Data deposited in or computed by PubChem | |
Molecular Formula |
C30H63Si | |
Source | PubChem | |
URL | https://pubchem.ncbi.nlm.nih.gov | |
Description | Data deposited in or computed by PubChem | |
DSSTOX Substance ID |
DTXSID90400196 | |
Record name | Tridecylsilane; | |
Source | EPA DSSTox | |
URL | https://comptox.epa.gov/dashboard/DTXSID90400196 | |
Description | DSSTox provides a high quality public chemistry resource for supporting improved predictive toxicology. | |
Molecular Weight |
451.9 g/mol | |
Source | PubChem | |
URL | https://pubchem.ncbi.nlm.nih.gov | |
Description | Data deposited in or computed by PubChem | |
CAS No. |
18765-73-6 | |
Record name | Tridecylsilane; | |
Source | EPA DSSTox | |
URL | https://comptox.epa.gov/dashboard/DTXSID90400196 | |
Description | DSSTox provides a high quality public chemistry resource for supporting improved predictive toxicology. | |
Retrosynthesis Analysis
AI-Powered Synthesis Planning: Our tool employs the Template_relevance Pistachio, Template_relevance Bkms_metabolic, Template_relevance Pistachio_ringbreaker, Template_relevance Reaxys, Template_relevance Reaxys_biocatalysis model, leveraging a vast database of chemical reactions to predict feasible synthetic routes.
One-Step Synthesis Focus: Specifically designed for one-step synthesis, it provides concise and direct routes for your target compounds, streamlining the synthesis process.
Accurate Predictions: Utilizing the extensive PISTACHIO, BKMS_METABOLIC, PISTACHIO_RINGBREAKER, REAXYS, REAXYS_BIOCATALYSIS database, our tool offers high-accuracy predictions, reflecting the latest in chemical research and data.
Strategy Settings
Precursor scoring | Relevance Heuristic |
---|---|
Min. plausibility | 0.01 |
Model | Template_relevance |
Template Set | Pistachio/Bkms_metabolic/Pistachio_ringbreaker/Reaxys/Reaxys_biocatalysis |
Top-N result to add to graph | 6 |
Feasible Synthetic Routes
Disclaimer and Information on In-Vitro Research Products
Please be aware that all articles and product information presented on BenchChem are intended solely for informational purposes. The products available for purchase on BenchChem are specifically designed for in-vitro studies, which are conducted outside of living organisms. In-vitro studies, derived from the Latin term "in glass," involve experiments performed in controlled laboratory settings using cells or tissues. It is important to note that these products are not categorized as medicines or drugs, and they have not received approval from the FDA for the prevention, treatment, or cure of any medical condition, ailment, or disease. We must emphasize that any form of bodily introduction of these products into humans or animals is strictly prohibited by law. It is essential to adhere to these guidelines to ensure compliance with legal and ethical standards in research and experimentation.