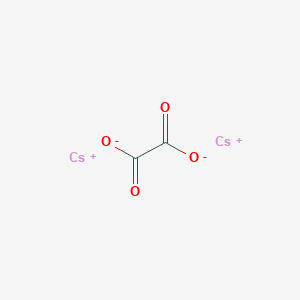
Cerium oxalate
- Click on QUICK INQUIRY to receive a quote from our team of experts.
- With the quality product at a COMPETITIVE price, you can focus more on your research.
Overview
Mechanism of Action
Target of Action
Cerium Oxalate, a compound of cerium, primarily targets the digestive system . It has been historically used as an antiemetic , especially in cases of pregnancy-related vomiting and motion sickness .
Mode of Action
It’s known that cerium, as a member of the lanthanide series, exerts diverse biological effects mainly by their resemblance to calcium . This similarity enables these elements to replace calcium in biomolecules without necessarily substituting for it functionally .
Biochemical Pathways
Cerium compounds have been shown to inhibit calcium-dependent physiological processes . These processes include those involved in the blood clotting cascade as well as in neuronal and muscular functions .
Pharmacokinetics
Research on cerium oxide nanoparticles, which have attracted attention in the pharmaceutical industry, suggests that understanding their biodistribution in the body is crucial for assessing potential toxicity .
Result of Action
Exposure to cerium salts can cause sensitivity to heat and increase the blood coagulation rate . Oxalates, including this compound, are known to be corrosive to tissue and powerful irritants, having a caustic effect on the linings of the digestive tracts and potentially causing kidney damage .
Action Environment
It’s worth noting that the environmental fate of cerium compounds, such as cerium dioxide nanoparticles, is highly dependent on their physicochemical properties .
Biochemical Analysis
Biochemical Properties
Cerium oxalate interacts with various biomolecules. For instance, it can react with oxalic acid to form cerium (III) chloride
Cellular Effects
This compound has been found to have effects on various types of cells. For example, cerium oxide nanoparticles, which can be synthesized from this compound, have shown promise as catalytic antioxidants in cell culture models . They exhibit redox activity, free radical scavenging property, and biofilm inhibition .
Molecular Mechanism
It is known that cerium (IV) can oxidize oxalic acid in a sulfuric acid medium . This reaction involves the formation of intermediate complexes of cerium (IV) with oxalic acid anions .
Temporal Effects in Laboratory Settings
It is known that this compound can be synthesized using the oxalate co-precipitation method, yielding single-phase oxalate precursors .
Dosage Effects in Animal Models
Cerium oxide nanoparticles, which can be synthesized from this compound, have shown promise as antioxidant and radioprotective agents in animal models of disease .
Metabolic Pathways
It is known that oxalic acid, a component of this compound, is involved in various metabolic processes in fungi and bacteria .
Transport and Distribution
Cerium oxide nanoparticles, which can be synthesized from this compound, have shown promise for their excellent pharmacokinetics and stable anti-oxidative activity .
Subcellular Localization
Cerium oxide nanoparticles, which can be synthesized from this compound, have shown promise for their unique electronic, optical, magnetic, and mechanical properties .
Preparation Methods
a. Synthetic Routes: Cerium oxalate can be synthesized by reacting oxalic acid (H₂C₂O₄) with cerium (III) chloride (CeCl₃). The balanced chemical equation for this reaction is:
3CeCl3+6H2C2O4→Ce2(C2O4)3+6HCl
b. Reaction Conditions: The reaction typically occurs in aqueous solution under mild conditions. Precipitation of this compound occurs upon mixing the reactants.
c. Industrial Production: Industrial production methods involve scaling up the synthesis process. Large-scale production may use continuous stirred tank reactors or other suitable equipment.
Chemical Reactions Analysis
Cerium oxalate participates in various chemical reactions:
Oxidation-Reduction Reactions: Cerium (III) ions can undergo redox reactions, transitioning between Ce³⁺ and Ce⁴⁺ states.
Substitution Reactions: this compound can exchange oxalate ligands with other anions or molecules.
Common Reagents and Conditions: Oxalic acid, cerium salts, and water are common reagents. Reaction conditions include mild temperatures and aqueous environments.
Major Products: The primary product is this compound itself.
Scientific Research Applications
Cerium oxalate finds applications in various fields:
Chemistry: Used as a precursor for cerium oxide (CeO₂) nanoparticles.
Biology: Investigated for potential antioxidant properties.
Medicine: Studied for its role in drug delivery systems.
Industry: Used in catalysts, ceramics, and glass polishing.
Comparison with Similar Compounds
Cerium oxalate stands out due to its unique combination of cerium ions and oxalate ligands. Similar compounds include other lanthanide oxalates (e.g., praseodymium oxalate, neodymium oxalate).
Properties
CAS No. |
139-42-4 |
---|---|
Molecular Formula |
C6Ce2O12 |
Molecular Weight |
544.29 g/mol |
IUPAC Name |
cerium(3+);oxalate |
InChI |
InChI=1S/3C2H2O4.2Ce/c3*3-1(4)2(5)6;;/h3*(H,3,4)(H,5,6);;/q;;;2*+3/p-6 |
InChI Key |
ZMZNLKYXLARXFY-UHFFFAOYSA-H |
SMILES |
C(=O)(C(=O)[O-])[O-].C(=O)(C(=O)[O-])[O-].C(=O)(C(=O)[O-])[O-].[Ce+3].[Ce+3] |
Canonical SMILES |
C(=O)(C(=O)[O-])[O-].C(=O)(C(=O)[O-])[O-].C(=O)(C(=O)[O-])[O-].[Ce+3].[Ce+3] |
Key on ui other cas no. |
1068-63-9 139-42-4 |
physical_description |
Commercial product contains oxalates of cerium, lanthanum, and other elements; Nonahydrate: White to pink solid, insoluble in water, soluble in hot acids; [Merck Index] |
Pictograms |
Irritant |
Origin of Product |
United States |
Retrosynthesis Analysis
AI-Powered Synthesis Planning: Our tool employs the Template_relevance Pistachio, Template_relevance Bkms_metabolic, Template_relevance Pistachio_ringbreaker, Template_relevance Reaxys, Template_relevance Reaxys_biocatalysis model, leveraging a vast database of chemical reactions to predict feasible synthetic routes.
One-Step Synthesis Focus: Specifically designed for one-step synthesis, it provides concise and direct routes for your target compounds, streamlining the synthesis process.
Accurate Predictions: Utilizing the extensive PISTACHIO, BKMS_METABOLIC, PISTACHIO_RINGBREAKER, REAXYS, REAXYS_BIOCATALYSIS database, our tool offers high-accuracy predictions, reflecting the latest in chemical research and data.
Strategy Settings
Precursor scoring | Relevance Heuristic |
---|---|
Min. plausibility | 0.01 |
Model | Template_relevance |
Template Set | Pistachio/Bkms_metabolic/Pistachio_ringbreaker/Reaxys/Reaxys_biocatalysis |
Top-N result to add to graph | 6 |
Feasible Synthetic Routes
Q1: What is the molecular formula and weight of cerium oxalate?
A1: this compound typically exists as a decahydrate with the molecular formula Ce2(C2O4)3·10H2O. Its molecular weight is 724.44 g/mol [, , , ].
Q2: What spectroscopic techniques are commonly used to characterize this compound?
A2: Several techniques are used to characterize this compound, including:
- X-ray diffraction (XRD): Confirms the monoclinic structure of Ce2(C2O4)3 and provides information about crystallinity [].
- Scanning electron microscopy (SEM): Reveals the morphology of this compound crystals, including size and shape [, , , , ].
- Energy-dispersive X-ray spectroscopy (EDS): Determines the elemental composition, confirming the presence of cerium, carbon, and oxygen [].
- Fourier-transform infrared spectroscopy (FTIR): Identifies functional groups and confirms the presence of water molecules and carboxylic groups [].
- Raman spectroscopy: Characterizes the vibrational modes of the compound, providing information about its structure and bonding [].
- Thermogravimetry (TG) and Differential thermal analysis (DTA): Used to study the thermal decomposition behavior of this compound [, , ].
Q3: How stable is this compound under different conditions?
A3: this compound decahydrate undergoes a multi-step thermal decomposition process, losing water molecules and decomposing to cerium oxide (CeO2) at higher temperatures [, , , ]. The stability and decomposition pathway can be influenced by factors like heating rate and atmosphere [].
Q4: What are the key factors influencing the morphology of this compound crystals during precipitation?
A4: Factors like temperature, concentration, acidity, and the addition rate of reactants (strike) during precipitation significantly influence the morphology of this compound crystals []. These parameters affect nucleation and crystal growth, leading to variations in crystal size and shape.
Q5: What is the role of this compound as a precursor material?
A5: this compound serves as a precursor for synthesizing cerium oxide (CeO2) nanoparticles, a technologically important material with applications in catalysis and solid oxide fuel cells [, , , , ].
Q6: How does the morphology of this compound impact the properties of the resulting cerium oxide?
A6: The morphology of this compound crystals significantly influences the properties of the final CeO2 nanoparticles []. For example, thinner this compound microcrystals tend to produce smaller CeO2 nanocrystals after calcination due to differences in diffusion limitations during grain growth.
Q7: What are the advantages of using this compound as a precursor for CeO2 compared to other methods?
A7: this compound decomposition offers several advantages for CeO2 synthesis:
- Controllable morphology: Different morphologies of CeO2 can be achieved by adjusting the precipitation conditions of this compound [].
- High surface area: Decomposition of this compound can lead to the formation of CeO2 with a high surface area, making it suitable for catalytic applications [].
- Relatively low temperatures: Compared to some other methods, CeO2 synthesis from this compound can be achieved at relatively low temperatures [].
Q8: What are the applications of this compound in research?
A8: this compound finds applications in various research areas, including:
- Simulating plutonium chemistry: Due to similarities in their chemical behavior, cerium is often used as a non-radioactive substitute for plutonium in studies related to nuclear waste reprocessing and precipitation processes [].
- Investigating non-classical crystallization: The precipitation of this compound serves as a model system for studying non-classical crystallization pathways, which involve transient amorphous phases and liquid droplets [, ].
- Developing novel phosphors: this compound formate, a hybrid inorganic-organic framework compound, has been investigated for its potential use in solid-state lighting applications due to its interesting optical properties and high mechanical strength [].
Q9: Are there any known biomedical applications or studies involving this compound?
A9: While historically this compound was explored for its potential antiemetic properties, research in this area is limited, and its use for such purposes is not currently prevalent [, , ]. It is crucial to consult with a healthcare professional before considering any substance for medicinal use.
Disclaimer and Information on In-Vitro Research Products
Please be aware that all articles and product information presented on BenchChem are intended solely for informational purposes. The products available for purchase on BenchChem are specifically designed for in-vitro studies, which are conducted outside of living organisms. In-vitro studies, derived from the Latin term "in glass," involve experiments performed in controlled laboratory settings using cells or tissues. It is important to note that these products are not categorized as medicines or drugs, and they have not received approval from the FDA for the prevention, treatment, or cure of any medical condition, ailment, or disease. We must emphasize that any form of bodily introduction of these products into humans or animals is strictly prohibited by law. It is essential to adhere to these guidelines to ensure compliance with legal and ethical standards in research and experimentation.