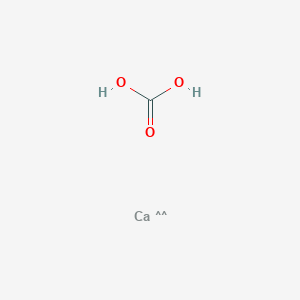
Aragonite (Ca(CO3)) (9CI)
Overview
Description
Aragonite, a metastable polymorph of calcium carbonate (CaCO₃), is distinguished by its orthorhombic crystal structure and high-pressure stability . Industrially, aragonite is prized for its needle-like morphology and superior mechanical properties, making it a preferred filler in paper, plastics, and biomedical applications . Unlike its more abundant polymorph calcite, aragonite forms under specific conditions, such as high Mg/Ca ratios in marine environments or elevated pressures . Its synthesis often involves controlled precipitation methods, where parameters like pH, temperature, and ion concentrations dictate polymorph selectivity .
Preparation Methods
Synthetic Routes and Reaction Conditions
Aragonite can be synthesized through various methods, including the carbonation process, where calcium hydroxide reacts with carbon dioxide under controlled conditions . Another method involves the precipitation of calcium carbonate from seawater, which can be optimized by adjusting factors such as temperature, pH, and the presence of biomolecules . The use of aspartic acid, for example, has been shown to influence the structure and morphology of the precipitated aragonite .
Industrial Production Methods
Industrial production of aragonite often involves the precipitation of calcium carbonate from solutions containing calcium ions and carbonate ions. This can be achieved through the carbonation process, where carbon dioxide is bubbled through a calcium hydroxide solution . The conditions, such as temperature and concentration, are carefully controlled to favor the formation of aragonite over other polymorphs like calcite .
Chemical Reactions Analysis
Reaction with Acids
Aragonite reacts with acids to release carbon dioxide, water, and calcium ions. This reaction underpins its role in neutralizing acidic environments:
-
Kinetics : Reactivity depends on acid strength and surface area. Concentrated hydrochloric acid induces rapid effervescence, while weaker acids (e.g., acetic acid) react more slowly .
-
Applications : Used in antacids and industrial acid neutralization .
Thermal Decomposition
Above 400°C, aragonite undergoes calcination to form calcium oxide (quicklime):
-
Stability : Aragonite decomposes at lower temperatures (~380°C) compared to calcite (~840°C) due to its metastable structure .
-
Byproducts : High-purity CO₂ gas is released, utilized in industrial processes like cement production .
Solubility in Water and CO₂
Aragonite’s solubility in water is governed by pH and CO₂ partial pressure (Pₛ꜀ₒ₂):
Pₛ꜀ₒ₂ (atm) | pH | [Ca²⁺] (mg/L) | Solubility Behavior |
---|---|---|---|
10⁻³.5 | 8.3 | 47 | Maximum solubility at ambient CO₂ |
10⁻¹² | 12.1 | 1200 | Forms Ca(OH)₂ due to low CO₂ |
10⁻¹ | 6.8 | 220 | Enhanced solubility from HCO₃⁻ |
-
Mechanism : Dissolves via:
This reaction contributes to karst formation and hard water .
Ion Exchange and Replacement Reactions
Aragonite participates in metal ion exchange, particularly with Pb²⁺ and Cd²⁺, forming less soluble carbonates:
Reaction with Pb²⁺
-
Kinetics : Faster replacement in aragonite than calcite due to structural similarity to cerussite (PbCO₃) .
Reaction with Cd²⁺
Comparative Reactivity with Calcite
Property | Aragonite | Calcite |
---|---|---|
Structure | Orthorhombic | Trigonal |
Reactivity with Pb²⁺ | Faster (morphological similarity) | Slower |
Thermal Stability | Decomposes at ~380°C | Decomposes at ~840°C |
Solubility in H₂O | Slightly higher | Slightly lower |
-
Mechanistic Insight : Aragonite’s ninefold oxygen coordination facilitates ion exchange, while calcite’s sixfold coordination resists substitution .
Phase Transformation
Aragonite transforms to calcite under hydrothermal conditions or prolonged exposure to water:
-
Activation Energy : ~150 kJ/mol, influenced by Mg²⁺ inhibition .
-
Environmental Impact : Affects sediment diagenesis and fossil preservation .
Interaction with Organics
Biological macromolecules (e.g., proteins, polyamines) stabilize aragonite in shells and pearls by:
This synthesis integrates structural, thermodynamic, and kinetic data to elucidate aragonite’s reactivity, emphasizing its role in geochemical, industrial, and biological systems .
Scientific Research Applications
Biomedical Applications
1.1 Drug Delivery Systems
Aragonite has been explored as a drug delivery agent due to its biocompatibility and biodegradability. A study demonstrated that surface-functionalized aragonite nanoparticles derived from cockle shells exhibited enhanced drug-loading capabilities and sustained release profiles. The nanoparticles showed improved cytotoxicity towards osteoblast cells, indicating their potential for targeted drug delivery in cancer therapy and other medical applications .
Key Findings:
- Size and Morphology: Aragonite nanoparticles can be engineered to achieve sizes below 100 nm, which is optimal for drug delivery.
- Surface Functionalization: Enhances dispersion and stability in biological environments.
- Biocompatibility: Demonstrated cytotoxicity improvement suggests suitability for biomedical applications.
1.2 Bone Tissue Engineering
The mechanical strength and bioactive properties of aragonite make it an excellent candidate for bone tissue engineering. Its ability to mimic the mineral composition of bone allows for effective integration into biological systems. Studies have shown that aragonite-based scaffolds support osteoconductivity and promote bone regeneration .
Environmental Applications
2.1 Carbon Capture and Storage (CCS)
Aragonite plays a role in carbon capture technologies due to its ability to sequester carbon dioxide (CO₂). Research indicates that aragonite can be synthesized from CO₂ in aqueous solutions, thus contributing to efforts aimed at reducing greenhouse gas emissions .
Key Findings:
- Synthesis Method: Aragonite can be produced through carbonation processes involving calcium hydroxide and sodium carbonate.
- Environmental Impact: Utilization of aragonite in CCS contributes to sustainable practices in industrial processes.
Industrial Applications
3.1 Inorganic Fillers
Aragonite is utilized as an inorganic filler in various materials to enhance mechanical properties. High aspect ratio aragonite whiskers are particularly effective in reinforcing polymers and composites, improving their strength and durability .
Key Findings:
- Mechanical Enhancement: Incorporation of aragonite whiskers significantly increases tensile strength and impact resistance of polymer matrices.
- Cost-Effectiveness: Using aragonite as a filler can reduce material costs while enhancing performance.
3.2 Precipitated Calcium Carbonate (PCC)
Aragonite is also synthesized as precipitated calcium carbonate (PCC) for use in coatings, plastics, and paper industries. The unique morphology of aragonite PCC provides superior performance characteristics compared to traditional calcite-based fillers .
Case Studies
Mechanism of Action
The mechanism by which aragonite exerts its effects is primarily related to its crystal structure and chemical composition. The orthorhombic structure of aragonite allows it to interact with various ions and molecules in its environment, influencing processes such as biomineralization and carbon sequestration . The presence of calcium ions in aragonite makes it a crucial component in biological systems, where it contributes to the formation of shells and skeletons in marine organisms .
Comparison with Similar Compounds
Crystal Structure and Coordination
Aragonite exhibits a hexagonal closest packing (HCP) arrangement with Ca²⁺ coordinated by nine oxygen atoms ([CaO₉] polyhedra), creating a layered structure parallel to the (001) plane . In contrast:
- Calcite (rhombohedral) has a cubic closest packing (CCP) structure with six-coordinate Ca²⁺ ([CaO₆] octahedra) .
- Vaterite (hexagonal) features a less ordered structure with alternating layers of Ca²⁺ and CO₃²⁻, often forming spherical aggregates .
Table 1: Structural Comparison of CaCO₃ Polymorphs
Property | Aragonite | Calcite | Vaterite |
---|---|---|---|
Crystal System | Orthorhombic | Rhombohedral | Hexagonal |
Coordination (Ca²⁺) | 9 | 6 | 6–8 |
Density (g/cm³) | 2.93–2.95 | 2.71–2.94 | 2.54–2.65 |
Common Morphology | Needles/Prisms | Rhombohedra | Spherulites |
Phase Transitions and High-Pressure Behavior
Under high pressure (>1.5 GPa), aragonite undergoes structural deformation, with [CO₃]²⁻ units rotating or displacing to form high-pressure polymorphs like CaCO₃-III or post-aragonite phases . These transitions involve overcoming kinetic barriers due to steric hindrance between CO₃²⁻ groups . Calcite, however, transforms to CaCO₃-II at ~1.5 GPa, retaining its CaO₆ coordination .
Contradictions and Uncertainties
Biological Activity
Aragonite, a polymorph of calcium carbonate (CaCO3), plays a significant role in biological systems, particularly in marine environments. This article delves into the biological activity of aragonite, exploring its formation mechanisms, interactions with biological entities, and implications for marine ecosystems.
Overview of Aragonite
Aragonite is characterized by its orthorhombic crystal structure and is commonly found in marine organisms such as corals and mollusks. Its biological significance stems from its role in biomineralization processes, where organisms utilize aragonite for structural support and protection.
Mechanisms of Aragonite Formation
The formation of aragonite in biological systems is influenced by various factors, including ionic composition, pH levels, and the presence of biomolecules. Key studies have highlighted the following mechanisms:
- Biomineralization by Coral Cells : Research on Pocillopora damicornis demonstrates that aragonite crystallization can occur in primary cell cultures. The study showed a transient increase in alkaline phosphatase (ALP) activity, which correlated with aragonite precipitation. Modifying the ionic formulation of the medium affected both ALP activation and aragonite precipitation timing .
- Bacterial Mediation : Bacteria such as Bacillus licheniformis play a crucial role in the incorporation of magnesium ions (Mg²⁺) into aragonite. Studies indicate that higher Mg²⁺ concentrations enhance aragonite formation through bacterial metabolic processes that increase local pH and carbonate alkalinity . The presence of extracellular polymeric substances (EPS) from bacteria aids in ion absorption, creating conditions favorable for aragonite nucleation .
Biological Interactions and Implications
Aragonite's biological activity extends beyond mere structural formation. It influences various ecological interactions:
- Coral Reef Formation : Aragonite is fundamental to coral reef ecosystems. The ability of corals to secrete aragonite is essential for reef-building processes. Variations in seawater chemistry, particularly Mg/Ca ratios, affect the prevalence of aragonite-forming species .
- Fluorine Incorporation : Recent studies suggest that aragonite can incorporate fluorine more readily than calcite. This incorporation may serve as an indicator of past oceanic conditions and can influence the biological responses of marine organisms to changing environmental parameters .
1. Coral Biomineralization
A study focusing on octocorals examined the evolutionary aspects of calcification and biomineralization processes. It highlighted gaps in understanding how different species utilize aragonite versus calcite for skeletal formation, suggesting potential research avenues to explore molecular mechanisms further .
2. Experimental Conditions
Research conducted under varying seawater conditions demonstrated that certain hypercalcifying organisms could only thrive during specific periods favoring either calcite or aragonite seas. This indicates a direct link between environmental chemistry and biological activity related to aragonite formation .
Data Tables
Research Findings
Recent findings emphasize the complexity of aragonite's role in biology:
- Influence of Biomolecules : The presence of specific amino acids and organic compounds enhances Mg²⁺ incorporation into aragonite crystals, thereby influencing their structural properties and stability .
- Environmental Sensitivity : The ability of marine organisms to adapt their mineralization processes based on environmental changes underscores the ecological significance of aragonite .
Q & A
Basic Research Questions
Q. How can aragonite be distinguished from calcite in laboratory settings, and what analytical methods are most effective for polymorph identification?
Aragonite and calcite share the same chemical formula (CaCO₃) but differ in crystal symmetry (orthorhombic vs. trigonal) and physical properties. Key distinguishing methods include:
- X-ray diffraction (XRD) : Aragonite exhibits distinct peaks at ~3.40 Å (d-spacing) for the (111) plane, while calcite shows a prominent peak at ~3.03 Å (104) .
- Raman spectroscopy : Aragonite has a strong ν₁ symmetric stretching mode at ~1,085 cm⁻¹, split into doublets due to its orthorhombic structure, unlike calcite’s single peak .
- Hardness and cleavage : Aragonite (Mohs 3.5–4) shows poor cleavage and conchoidal fracture, contrasting with calcite’s perfect rhombohedral cleavage and lower hardness (Mohs 3) .
Q. What experimental conditions favor the precipitation of aragonite over calcite in synthetic systems?
Aragonite formation is kinetically favored under:
- Elevated Mg/Ca ratios : Mg²⁺ inhibits calcite nucleation by adsorbing onto growth sites, promoting metastable aragonite precipitation. Solutions with Mg/Ca > 2:1 are effective .
- High CO₂ partial pressure (pCO₂) : Controlled degassing experiments (e.g., 20,000 ppmV pCO₂) mimic natural cave environments, stabilizing aragonite via rapid CO₂ outgassing and increased carbonate saturation .
- Trace element additives : Sr²⁺ or Pb²⁺ substitution in the CaCO₃ lattice (e.g., >7.7% Pb²⁺) stabilizes aragonite-type solid solutions by distorting the crystal structure .
Advanced Research Questions
Q. How do boron partitioning mechanisms in aragonite vary with fluid chemistry, and what implications do these have for paleo-pH reconstructions?
Boron incorporation into aragonite (B/Ca ratio) is governed by:
- Adsorption vs. lattice substitution : B(OH)₄⁻ adsorption onto crystal surfaces dominates at low pH, while substitution for CO₃²⁻ occurs at higher pH. This pH dependency complicates KD (partition coefficient) calculations .
- Fluid [CO₃²⁻] : A positive correlation exists between [CO₃²⁻] and B/Ca ratios in abiogenic aragonite, validated by experimental models (e.g., Holcomb et al. 2016: KD = 0.0013 × [CO₃²⁻] + 0.017) .
- Sodium co-precipitation : Charge balance via Na⁺ incorporation can alter B partitioning, requiring corrections in paleo-pH proxies .
Q. What thermodynamic and kinetic factors control the aragonite-calcite phase transition at high pressures, and how can these be modeled experimentally?
- High-pressure stability : Aragonite is thermodynamically stable above ~3 GPa at 25°C, but metastable persistence at lower pressures is common due to kinetic barriers. In situ Raman spectroscopy reveals pressure-induced lattice compression trends (e.g., shifts in ν₁ CO₃²⁻ modes) .
- Solid-solution models : Guggenheim coefficients (e.g., a₀ = 2.94 for (Ca,Pb)CO₃) quantify non-ideal mixing in solid solutions, critical for predicting phase boundaries .
- Hydrous vs. anhydrous systems : Water reduces activation energy for calcite nucleation, accelerating aragonite-to-calcite transformation in hydrous experiments .
Q. How can calcium isotope fractionation (Δ⁴⁴/⁴⁰Ca) in aragonite be calibrated for paleoenvironmental studies, and what experimental designs minimize kinetic biases?
- Cave-analogue experiments : Tightly controlled setups (e.g., constant drip rates, thin solution films) replicate natural speleothem growth, yielding Δ⁴⁴/⁴⁰Ca of −1.5‰ to −0.5‰ relative to fluid, with temperature dependence of ~0.02‰/°C .
- Ionic strength effects : Low ionic strength solutions (<0.1 M) minimize kinetic fractionation, as high ionic strength enhances ion hydration and non-equilibrium isotope effects .
- Multi-element doping : Adding Sr²⁺ or Mg²⁺ at natural concentrations (e.g., Sr/Ca ~1 mmol/mol) ensures isotopic behavior mirrors biogenic aragonite .
Q. Methodological Recommendations
- For isotopic studies : Use cave-analogue setups with controlled drip rates and CO₂ degassing to replicate natural growth kinetics .
- For paleo-pH proxies : Account for fluid [CO₃²⁻] and Na⁺ co-precipitation when interpreting B/Ca ratios in biogenic aragonite .
- For phase stability models : Incorporate Guggenheim coefficients and hydrous/anhydrous boundary conditions to refine thermodynamic predictions .
Properties
InChI |
InChI=1S/CH2O3.Ca/c2-1(3)4;/h(H2,2,3,4); | |
---|---|---|
Source | PubChem | |
URL | https://pubchem.ncbi.nlm.nih.gov | |
Description | Data deposited in or computed by PubChem | |
InChI Key |
FYHXNYLLNIKZMR-UHFFFAOYSA-N | |
Source | PubChem | |
URL | https://pubchem.ncbi.nlm.nih.gov | |
Description | Data deposited in or computed by PubChem | |
Canonical SMILES |
C(=O)(O)O.[Ca] | |
Source | PubChem | |
URL | https://pubchem.ncbi.nlm.nih.gov | |
Description | Data deposited in or computed by PubChem | |
Molecular Formula |
CH2CaO3 | |
Source | PubChem | |
URL | https://pubchem.ncbi.nlm.nih.gov | |
Description | Data deposited in or computed by PubChem | |
DSSTOX Substance ID |
DTXSID80163829 | |
Record name | Aragonite | |
Source | EPA DSSTox | |
URL | https://comptox.epa.gov/dashboard/DTXSID80163829 | |
Description | DSSTox provides a high quality public chemistry resource for supporting improved predictive toxicology. | |
Molecular Weight |
102.10 g/mol | |
Source | PubChem | |
URL | https://pubchem.ncbi.nlm.nih.gov | |
Description | Data deposited in or computed by PubChem | |
CAS No. |
471-34-1, 3983-19-5, 14791-73-2, 13397-26-7 | |
Record name | Calcium carbonate | |
Source | CAS Common Chemistry | |
URL | https://commonchemistry.cas.org/detail?cas_rn=471-34-1 | |
Description | CAS Common Chemistry is an open community resource for accessing chemical information. Nearly 500,000 chemical substances from CAS REGISTRY cover areas of community interest, including common and frequently regulated chemicals, and those relevant to high school and undergraduate chemistry classes. This chemical information, curated by our expert scientists, is provided in alignment with our mission as a division of the American Chemical Society. | |
Explanation | The data from CAS Common Chemistry is provided under a CC-BY-NC 4.0 license, unless otherwise stated. | |
Record name | Calcium bicarbonate | |
Source | CAS Common Chemistry | |
URL | https://commonchemistry.cas.org/detail?cas_rn=3983-19-5 | |
Description | CAS Common Chemistry is an open community resource for accessing chemical information. Nearly 500,000 chemical substances from CAS REGISTRY cover areas of community interest, including common and frequently regulated chemicals, and those relevant to high school and undergraduate chemistry classes. This chemical information, curated by our expert scientists, is provided in alignment with our mission as a division of the American Chemical Society. | |
Explanation | The data from CAS Common Chemistry is provided under a CC-BY-NC 4.0 license, unless otherwise stated. | |
Record name | Aragonite | |
Source | CAS Common Chemistry | |
URL | https://commonchemistry.cas.org/detail?cas_rn=14791-73-2 | |
Description | CAS Common Chemistry is an open community resource for accessing chemical information. Nearly 500,000 chemical substances from CAS REGISTRY cover areas of community interest, including common and frequently regulated chemicals, and those relevant to high school and undergraduate chemistry classes. This chemical information, curated by our expert scientists, is provided in alignment with our mission as a division of the American Chemical Society. | |
Explanation | The data from CAS Common Chemistry is provided under a CC-BY-NC 4.0 license, unless otherwise stated. | |
Record name | Calcite | |
Source | CAS Common Chemistry | |
URL | https://commonchemistry.cas.org/detail?cas_rn=13397-26-7 | |
Description | CAS Common Chemistry is an open community resource for accessing chemical information. Nearly 500,000 chemical substances from CAS REGISTRY cover areas of community interest, including common and frequently regulated chemicals, and those relevant to high school and undergraduate chemistry classes. This chemical information, curated by our expert scientists, is provided in alignment with our mission as a division of the American Chemical Society. | |
Explanation | The data from CAS Common Chemistry is provided under a CC-BY-NC 4.0 license, unless otherwise stated. | |
Record name | Aragonite | |
Source | ChemIDplus | |
URL | https://pubchem.ncbi.nlm.nih.gov/substance/?source=chemidplus&sourceid=0014791732 | |
Description | ChemIDplus is a free, web search system that provides access to the structure and nomenclature authority files used for the identification of chemical substances cited in National Library of Medicine (NLM) databases, including the TOXNET system. | |
Record name | Aragonite | |
Source | EPA DSSTox | |
URL | https://comptox.epa.gov/dashboard/DTXSID80163829 | |
Description | DSSTox provides a high quality public chemistry resource for supporting improved predictive toxicology. | |
Retrosynthesis Analysis
AI-Powered Synthesis Planning: Our tool employs the Template_relevance Pistachio, Template_relevance Bkms_metabolic, Template_relevance Pistachio_ringbreaker, Template_relevance Reaxys, Template_relevance Reaxys_biocatalysis model, leveraging a vast database of chemical reactions to predict feasible synthetic routes.
One-Step Synthesis Focus: Specifically designed for one-step synthesis, it provides concise and direct routes for your target compounds, streamlining the synthesis process.
Accurate Predictions: Utilizing the extensive PISTACHIO, BKMS_METABOLIC, PISTACHIO_RINGBREAKER, REAXYS, REAXYS_BIOCATALYSIS database, our tool offers high-accuracy predictions, reflecting the latest in chemical research and data.
Strategy Settings
Precursor scoring | Relevance Heuristic |
---|---|
Min. plausibility | 0.01 |
Model | Template_relevance |
Template Set | Pistachio/Bkms_metabolic/Pistachio_ringbreaker/Reaxys/Reaxys_biocatalysis |
Top-N result to add to graph | 6 |
Feasible Synthetic Routes
Disclaimer and Information on In-Vitro Research Products
Please be aware that all articles and product information presented on BenchChem are intended solely for informational purposes. The products available for purchase on BenchChem are specifically designed for in-vitro studies, which are conducted outside of living organisms. In-vitro studies, derived from the Latin term "in glass," involve experiments performed in controlled laboratory settings using cells or tissues. It is important to note that these products are not categorized as medicines or drugs, and they have not received approval from the FDA for the prevention, treatment, or cure of any medical condition, ailment, or disease. We must emphasize that any form of bodily introduction of these products into humans or animals is strictly prohibited by law. It is essential to adhere to these guidelines to ensure compliance with legal and ethical standards in research and experimentation.