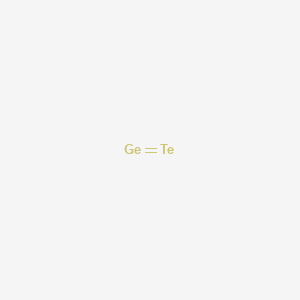
Germanium telluride (GeTe)
- Click on QUICK INQUIRY to receive a quote from our team of experts.
- With the quality product at a COMPETITIVE price, you can focus more on your research.
Overview
Description
Germanium telluride (GeTe) is a group-IV-VI compound with a narrow bandgap (~0.7 eV in bulk) and dual-phase crystallography: a rhombohedral (α-GeTe) structure at low temperatures and a cubic (β-GeTe) structure above 700 K . It exhibits exceptional thermoelectric (TE) performance, phase-change memory (PCM) capabilities, and ferroelectric properties. Key attributes include:
- Thermoelectric Efficiency: GeTe achieves a high figure of merit (zT) up to 2.3 via strategies like doping (Bi, In), nanostructuring, and vacancy engineering .
- Low Thermal Conductivity: Peierls distortion in the rhombohedral phase reduces lattice thermal conductivity to ~1.5–2.0 W/m·K .
- Phase-Change Applications: Reversible switching between amorphous and crystalline states enables use in optical data storage and non-volatile memory .
Preparation Methods
Synthetic Routes and Reaction Conditions: Germanium telluride (GeTe) can be synthesized through several methods. One common approach involves the high-temperature sintering of germanium and tellurium powders in a tube furnace. This process typically requires temperatures around 700°C to 800°C. Another method involves the chemical vapor deposition (CVD) technique, where germanium and tellurium precursors are reacted in a controlled environment to form thin films of germanium telluride .
Industrial Production Methods: In industrial settings, germanium telluride is often produced using melt spinning and hot pressing techniques. These methods involve melting the raw materials and rapidly cooling them to form fine-grained structures. The resulting material is then subjected to hot pressing to enhance its thermoelectric properties .
Chemical Reactions Analysis
Phase Transition Mechanisms
GeTe undergoes reversible amorphous-crystalline phase transitions driven by thermal or electrical stimuli:
2.1 Thermal Actuation
-
Amorphous → Crystalline : Heating to crystallization temperature (~200°C for bulk, ~250°C for nanoparticles) with gradual cooling .
-
Crystalline → Amorphous : Rapid quenching after melting (~725°C) .
2.2 Structural Dynamics
During crystallization, Ge-Ge homopolar bonds cleave, forming Te-Ge-Te "bridge" intermediates. This diffusionless process preserves the Te sublattice while Ge atoms rearrange into octahedral sites .
Phase | Resistivity | Structure |
---|---|---|
Amorphous | High (~10⁶ Ω·cm) | Disordered Ge chains |
Crystalline | Low (~10⁻³ Ω·cm) | Rhombohedral α-GeTe |
Doping and Alloying Reactions
3.1 p-Type Doping
GeTe inherently exhibits p-type conductivity due to Ge vacancies (V<sub>Ge</sub>). Alloying with AgInTe₂ increases V<sub>Ge</sub> concentration, stabilizing cubic GeTe but reducing carrier mobility .
3.2 n-Type Conversion
Heavy Bi doping introduces n-type conduction by compensating V<sub>Ge</sub> acceptors. Bi<sub>Ge</sub> defects raise the Fermi level, enabling electron-dominated transport .
Dopant | Effect | Conductivity |
---|---|---|
AgInTe₂ | ↑ V<sub>Ge</sub>, cubic stabilization | p-type |
Bi | ↓ V<sub>Ge</sub>, Fermi level shift | n-type |
Defect Chemistry
4.1 Ge Vacancies (V<sub>Ge</sub>)
-
Formation Energy : Lower in cubic GeTe than rhombohedral phase, favoring spontaneous vacancy generation .
-
Role : Act as shallow acceptors, contributing to persistent p-type conductivity (hole concentration ~10²¹ cm⁻³) .
4.2 Electronic Impact
V<sub>Ge</sub> modify the valence band maximum (VBM), reducing power factor in thermoelectric applications. Alloying with AgInTe₂ exacerbates this effect but suppresses lattice thermal conductivity .
Catalytic and Surface Reactions
GeTe monolayers (2D) demonstrate photocatalytic potential for water splitting due to:
Scientific Research Applications
Thermoelectric Applications
Germanium telluride has emerged as a leading candidate for thermoelectric materials due to its high thermoelectric performance.
- Performance Metrics : GeTe exhibits a high figure of merit (ZT), which measures the efficiency of thermoelectric materials. Recent studies have reported ZT values exceeding 2.14 at mid-temperatures when doped with transition metals like Molybdenum (Mo) and co-doped with elements such as Antimony (Sb) or Bismuth (Bi) .
- Mechanisms : The enhancement in thermoelectric performance is attributed to the reduction of thermal conductivity through microcrystalline-assisted grain boundary formations, which help maintain a low thermal conductivity while improving electrical properties .
Doping Element | Maximum ZT | Temperature (K) |
---|---|---|
Molybdenum | 2.14 | 673 |
Antimony | 2.30 | 673 |
Phase Change Memory and Data Storage
GeTe is widely recognized for its role in phase change memory (PCM) technologies.
- Phase Transition : GeTe undergoes rapid and reversible phase transitions between amorphous and crystalline states, which allows for significant changes in resistivity—up to six orders of magnitude . This property is exploited in data storage applications, enabling high-speed data writing and erasing.
- Case Study : Research has demonstrated that GeTe-based materials are integral to the development of rewritable optical media such as CDs and DVDs . The material's ability to switch states quickly makes it suitable for next-generation memory devices.
Radio Frequency Switching
The unique electrical properties of GeTe make it an excellent candidate for radio frequency (RF) switching applications.
- Performance Characteristics : GeTe films exhibit low insertion loss, high isolation, fast switching speeds, and low power consumption . These attributes are crucial for RF front-end systems and reconfigurable networks.
- Device Structures : Various device structures have been developed using GeTe films, including directly heated and indirectly heated RF phase-change switches. Each structure's performance is influenced by the intrinsic properties of the material layers used .
Photocatalytic Water Splitting
The two-dimensional monolayer form of germanium telluride has shown promise in photocatalytic applications.
- Band Gap Properties : The monolayer GeTe exhibits a band gap of approximately 2.35 eV, making it suitable for visible-light-driven photocatalytic water splitting . Its high electron and hole mobilities enhance its effectiveness as a photocatalyst.
- Research Findings : Density functional theory computations indicate that GeTe can effectively harvest solar energy for chemical reactions, positioning it as a potential candidate for sustainable energy solutions .
Optoelectronic Devices
GeTe's semiconducting properties lend themselves well to optoelectronic applications.
- Defect Engineering : Understanding the role of germanium vacancies within GeTe has been crucial for optimizing its performance in optoelectronic devices. Studies indicate that controlling these vacancies can improve the material's structural stability and transport properties .
- Applications : GeTe-based materials are being explored for use in various optoelectronic devices, including light-emitting diodes (LEDs) and lasers, due to their favorable electronic characteristics .
Mechanism of Action
The mechanism of action of germanium telluride is primarily based on its ability to undergo reversible phase transitions between amorphous and crystalline states. This transition is induced by external stimuli such as heat or electrical pulses. The crystalline state exhibits low resistivity, while the amorphous state has high resistivity. This property is exploited in data storage and switching applications .
At the molecular level, the phase transition involves the rearrangement of germanium and tellurium atoms, leading to significant changes in electrical and thermal properties. The ferroelectric polarization of germanium telluride can also be switched by external electric fields, making it useful in spintronic applications .
Comparison with Similar Compounds
Group-IV Tellurides: SnTe and PbTe
Key Insights :
- GeTe vs. SnTe : GeTe outperforms SnTe in zT due to lower thermal conductivity and higher Seebeck coefficients. SnTe’s higher hole concentration requires heavy doping for optimization .
- GeTe vs. PbTe : PbTe is a benchmark TE material but suffers from mechanical instability and lead toxicity. GeTe offers comparable zT with superior mechanical robustness .
Bismuth Telluride (Bi₂Te₃) and Antimony Telluride (Sb₂Te₃)
Key Insights :
- GeTe excels in medium-temperature (600–800 K) regimes .
- Sb₂Te₃ alloys (e.g., GeSbTe) are PCM competitors but trade stability for faster switching speeds compared to GeTe .
Cadmium Telluride (CdTe) and Phase-Change Alloys
Key Insights :
- CdTe is optoelectronically inert compared to GeTe but excels in radiation detection due to its wide bandgap .
- GST alloys (e.g., Ge₂Sb₂Te₅) exhibit faster crystallization than GeTe but lower thermal stability .
Research Advancements and Challenges
Doping and Nanostructuring
- Doping : Bi and In co-doping in GeTe reduces hole concentration and enhances zT to 2.3 .
- Nanocomposites: CoGe₂ and Co₅Ge₇ precipitates in GeTe improve electrical conductivity by 20% while suppressing thermal conductivity .
- Phase Stability : Indium alloying (e.g., InGeTe₂) enhances crystallization temperature for photonic applications .
Limitations
Biological Activity
Germanium telluride (GeTe) is a compound that has garnered attention for its unique properties and potential applications in various fields, including electronics and materials science. However, its biological activity remains an area of emerging research. This article aims to explore the biological implications of GeTe, particularly focusing on its interactions at the cellular level, potential therapeutic uses, and the mechanisms underlying its biological effects.
Overview of Germanium Telluride
Germanium telluride is a semiconductor material known for its phase-change properties, which have made it a candidate for applications in data storage and photonics. Its chemical formula is GeTe, and it belongs to the group of binary compounds formed from germanium and tellurium. The compound exhibits both metallic and polar characteristics, which contribute to its unique electrical properties.
Antioxidant Properties
Research has indicated that germanium compounds, including GeTe, may exhibit antioxidant activities. Antioxidants are crucial in mitigating oxidative stress within biological systems. In studies involving organic germanium compounds, it has been observed that these compounds can enhance the activity of various antioxidant enzymes, such as superoxide dismutase (SOD) and catalase (CAT) .
Table 1: Antioxidant Activity of Germanium Compounds
Compound | Effect on SOD Activity | Effect on CAT Activity | Reference |
---|---|---|---|
Ge-132 | Increased | Increased | |
Spirogermanium | Increased | Not specified | |
GeTe | Potentially increased | Not specified | Emerging research |
Immunomodulatory Effects
GeTe has shown potential immunomodulatory effects similar to other germanium compounds. For instance, studies have demonstrated that germanium can enhance immune responses by increasing interferon production and activating natural killer (NK) cells and macrophages . This suggests that GeTe may have applications in enhancing immune function or as an adjuvant in vaccine formulations.
Case Study: Immune Response Enhancement
- Study : Oral administration of Ge-132 in mice resulted in significant increases in serum interferon levels.
- Findings : Enhanced NK cell activity was observed alongside increased macrophage activation .
The biological activity of GeTe can be attributed to several mechanisms:
- Oxidative Stress Mitigation : By enhancing antioxidant enzyme activity, GeTe may help reduce oxidative damage in cells.
- Immune Activation : The compound appears to stimulate immune cells, leading to enhanced responses against pathogens.
- Cellular Uptake : Germanium compounds can enter cells through various transport mechanisms, allowing them to exert their effects intracellularly .
Toxicity and Safety Considerations
While germanium compounds can offer therapeutic benefits, it is essential to consider their toxicity profiles. Tellurium compounds are known to exhibit toxicity at certain concentrations, which raises concerns about the safety of GeTe . Research into the safe dosage levels and potential side effects is crucial for future applications.
Q & A
Basic Research Questions
Q. What experimental techniques are critical for characterizing the structural and electronic properties of GeTe?
Methodological Answer:
- X-ray Photoelectron Spectroscopy (XPS) is used to analyze stoichiometry and binding states, though overlapping binding energy (BE) peaks (e.g., Ge 3d and Te 4d) complicate interpretation, especially in air-exposed samples. Charge correction and complementary techniques like Extended X-ray Absorption Fine Structure (EXAFS) are recommended for resolving coordination environments .
- Modulated Photocurrent Technique and Photothermal Deflection Spectroscopy quantify the density of states (DOS) in amorphous GeTe films by correlating photoconductivity and absorption data with numerical simulations .
Q. How does annealing temperature influence the structural and optical properties of GeTe thin films?
Methodological Answer:
- Annealing induces crystallization, altering resistivity and optical bandgap. Key steps include:
Temperature-dependent resistivity measurements (e.g., in-situ heating stages) to track phase transitions (~200°C for amorphous-to-crystalline shifts) .
X-ray Diffraction (XRD) to identify structural phases (rhombohedral vs. cubic) and grain size evolution .
Spectroscopic Ellipsometry to measure optical constants (refractive index, extinction coefficient) across wavelengths .
Q. What parameters govern phase transition kinetics in GeTe-based phase-change memory (PCM) devices?
Methodological Answer:
- Pulse duration and amplitude in electrical/thermal stimulation control nucleation and growth rates .
- Time-resolved resistance measurements quantify transition speeds (nanosecond-scale crystallization) .
- In-situ TEM or XRD during heating/cooling cycles visualizes real-time structural dynamics .
Advanced Research Questions
Q. How can overlapping XPS spectra in nitrogen-doped GeTe alloys be resolved?
Methodological Answer:
- Use high-resolution synchrotron XPS with monochromatic sources to enhance peak separation.
- Apply multivariate curve resolution (MCR) or principal component analysis (PCA) to deconvolute overlapping Ge 3d and N 1s peaks .
- Validate with EXAFS to independently assess coordination environments and bond lengths .
Q. What computational methods predict GeTe nanocrystal morphology and ligand interactions?
Methodological Answer:
- Dispersion-corrected Density Functional Theory (DFT-D) simulates low-index facets (e.g., (100), (110)) and ligand adsorption energies (e.g., oleylamine, TOPO) to explain experimental shapes (nanoplates vs. spheres) .
- Wulff Construction models equilibrium morphologies using surface energies derived from DFT .
Q. How do resistance fluctuations probe aging effects in GeTe glass states?
Methodological Answer:
- In-situ resistance monitoring post-melt-quenching tracks power-law-like resistivity increases, correlating with structural relaxation .
- Noise spectroscopy analyzes low-frequency resistance fluctuations to quantify defect dynamics and aging timescales .
Q. What strategies address data contradictions from stoichiometric variations in GeTe films?
Methodological Answer:
- Combinatorial deposition creates compositionally graded libraries for high-throughput property mapping (e.g., resistivity, optical bandgap) .
- Atom Probe Tomography (APT) or EDS/TEM validates local stoichiometry, reducing uncertainties in bulk-averaged measurements .
Q. How does Mn doping enhance multiferroic properties in GeTe?
Methodological Answer:
- Magnetoelectric coupling is probed via:
SQUID Magnetometry to measure magnetic hysteresis.
Piezoresponse Force Microscopy (PFM) to map ferroelectric domains .
- Density Functional Theory (DFT) calculates spin-polarized band structures to explain Mn-induced Rashba effects .
Q. What in-situ methods observe structural dynamics during GeTe phase transitions?
Methodological Answer:
- Time-domain Terahertz Spectroscopy tracks conductivity changes in split-ring resonators during phase transitions .
- Raman Spectroscopy with a heating stage monitors phonon mode shifts during crystallization .
Q. How can DFT improve the design of GeTe-based thermoelectric materials?
Methodological Answer:
Properties
CAS No. |
12025-39-7 |
---|---|
Molecular Formula |
GeTe |
Molecular Weight |
200.2 g/mol |
IUPAC Name |
germanium;tellurium |
InChI |
InChI=1S/Ge.Te |
InChI Key |
VDDXNVZUVZULMR-UHFFFAOYSA-N |
SMILES |
[Ge]=[Te] |
Canonical SMILES |
[Ge].[Te] |
Key on ui other cas no. |
12025-39-7 |
Pictograms |
Irritant |
Origin of Product |
United States |
Disclaimer and Information on In-Vitro Research Products
Please be aware that all articles and product information presented on BenchChem are intended solely for informational purposes. The products available for purchase on BenchChem are specifically designed for in-vitro studies, which are conducted outside of living organisms. In-vitro studies, derived from the Latin term "in glass," involve experiments performed in controlled laboratory settings using cells or tissues. It is important to note that these products are not categorized as medicines or drugs, and they have not received approval from the FDA for the prevention, treatment, or cure of any medical condition, ailment, or disease. We must emphasize that any form of bodily introduction of these products into humans or animals is strictly prohibited by law. It is essential to adhere to these guidelines to ensure compliance with legal and ethical standards in research and experimentation.