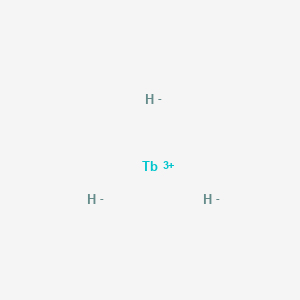
Terbium hydride
Overview
Description
Terbium hydride is a chemical compound consisting of terbium and hydrogen. Terbium is a rare earth metal belonging to the lanthanide series, and it forms hydrides when it reacts with hydrogen. This compound is known for its unique properties, including its potential superconductivity under high pressure and its role in enhancing the magnetic properties of certain materials .
Preparation Methods
Synthetic Routes and Reaction Conditions: Terbium hydride can be synthesized through the reaction of terbium chloride with lithium hydride. The reaction conditions are optimized to ensure the efficient formation of this compound. The resultant this compound can then be used to obtain metallic terbium through vacuum thermolysis .
Industrial Production Methods: In an industrial setting, this compound is typically produced by reacting terbium metal with hydrogen gas at elevated temperatures. This process involves heating terbium in a hydrogen atmosphere, leading to the formation of this compound. The reaction is usually carried out in a controlled environment to ensure the purity and stability of the product .
Types of Reactions:
Oxidation: this compound can undergo oxidation reactions, forming terbium oxide and releasing hydrogen gas.
Reduction: It can also participate in reduction reactions, where it acts as a reducing agent.
Substitution: this compound can undergo substitution reactions with other compounds, leading to the formation of various terbium-containing products.
Common Reagents and Conditions:
Oxidation: Typically involves exposure to oxygen or air at elevated temperatures.
Reduction: Often involves the use of reducing agents such as lithium aluminum hydride.
Substitution: Can involve various organic and inorganic reagents under controlled conditions.
Major Products Formed:
Terbium Oxide: Formed during oxidation reactions.
Terbium Salts: Formed during substitution reactions with acids.
Scientific Research Applications
Terbium hydride has several scientific research applications:
Magnetic Materials: It is used to enhance the magnetic properties of sintered magnets, particularly those based on neodymium-iron-boron compositions.
Superconductivity: this compound is being studied for its potential high-temperature superconductivity under high pressures.
Catalysis: It is used in catalytic processes, particularly in reactions involving hydrogenation and dehydrogenation.
Material Science: this compound is used in the synthesis of advanced materials with unique properties, such as high coercivity and stability.
Mechanism of Action
The mechanism by which terbium hydride exerts its effects is primarily related to its ability to donate hydrogen atoms in chemical reactions. This property makes it a valuable reducing agent and a participant in hydrogenation reactions. In magnetic materials, this compound contributes to the formation of a chemically nanoinhomogeneous structure, which enhances the magnetic properties of the material .
Comparison with Similar Compounds
Ytterbium Hydride: Similar to this compound, ytthis compound is also studied for its potential superconductivity and its role in enhancing magnetic properties.
Calcium Hydride: Used in similar applications, particularly in catalysis and hydrogen storage.
Uniqueness of this compound: this compound is unique due to its specific magnetic properties and its potential for high-temperature superconductivity. Its ability to form a chemically nanoinhomogeneous structure in magnetic materials sets it apart from other hydrides .
This compound continues to be a subject of extensive research due to its promising applications in various scientific and industrial fields.
Biological Activity
Terbium hydride (TbH) is a compound that has garnered interest in various fields, including materials science and biomedicine. This article focuses on its biological activity, exploring its potential applications and implications in medical and biological contexts.
Overview of this compound
This compound is formed when terbium, a rare earth metal, reacts with hydrogen. The most common form is TbH, where can vary depending on the conditions of synthesis and the specific application. The unique properties of terbium, including its luminescent characteristics and ability to form stable hydrides, make it a candidate for various biomedical applications.
1. Biocompatibility Studies
Recent studies have highlighted the biocompatibility of terbium-doped materials. For instance, research on terbium ions incorporated into hydroxyapatite (HAp) demonstrated that varying concentrations of terbium enhance cell viability and proliferation. The results indicated that Tb-doped HAp maintains its physicochemical properties while introducing new photoluminescent features beneficial for biomedical applications .
Concentration of Tb (x) | Cell Viability (%) | Photoluminescence Properties |
---|---|---|
0.05 | High | Moderate |
0.1 | High | Enhanced |
0.25 | Moderate | Strong |
0.5 | Moderate | Very Strong |
1 | Low | Saturated |
2. Drug Delivery Systems
Layered terbium hydroxides (LTbH) have been explored as platforms for drug delivery and fluorescence imaging. A study evaluated LTbH's ability to load nonsteroidal anti-inflammatory drugs (NSAIDs) such as diclofenac, ibuprofen, and naproxen. The findings revealed that these compounds could be rapidly released in physiological conditions (pH 7.4), indicating potential for therapeutic applications .
Key Findings:
- Rapid Drug Release : All formulations released their drug cargo within approximately 5 hours.
- Fluorescence Tracking : The fluorescence intensity varied based on the guest anion, allowing tracking of drug release.
Case Study 1: Terbium-Doped Hydroxyapatite
A study conducted by researchers focused on the synthesis of terbium-doped hydroxyapatite for biomedical applications. The results showed that increasing terbium concentration affected both the structural integrity and the biological response of the material:
- Methods : FTIR and X-ray diffraction were used to analyze structural changes.
- Results : Enhanced cell proliferation was observed with optimal doping levels, suggesting a balance between structural stability and biological activity.
Case Study 2: Layered Terbium Hydroxides in Drug Delivery
In another investigation, layered terbium hydroxides were tested for their drug delivery capabilities:
- Drug Loading : LTbH was loaded with NSAIDs through ion-exchange methods.
- Release Kinetics : The study demonstrated that drug release was rapid and dependent on the chemical nature of the guest anions.
Properties
IUPAC Name |
hydride;terbium(3+) | |
---|---|---|
Source | PubChem | |
URL | https://pubchem.ncbi.nlm.nih.gov | |
Description | Data deposited in or computed by PubChem | |
InChI |
InChI=1S/Tb.3H/q+3;3*-1 | |
Source | PubChem | |
URL | https://pubchem.ncbi.nlm.nih.gov | |
Description | Data deposited in or computed by PubChem | |
InChI Key |
WOLWRTFLUVVVTF-UHFFFAOYSA-N | |
Source | PubChem | |
URL | https://pubchem.ncbi.nlm.nih.gov | |
Description | Data deposited in or computed by PubChem | |
Canonical SMILES |
[H-].[H-].[H-].[Tb+3] | |
Source | PubChem | |
URL | https://pubchem.ncbi.nlm.nih.gov | |
Description | Data deposited in or computed by PubChem | |
Molecular Formula |
H3Tb | |
Source | PubChem | |
URL | https://pubchem.ncbi.nlm.nih.gov | |
Description | Data deposited in or computed by PubChem | |
Molecular Weight |
161.949 g/mol | |
Source | PubChem | |
URL | https://pubchem.ncbi.nlm.nih.gov | |
Description | Data deposited in or computed by PubChem | |
CAS No. |
13598-54-4 | |
Record name | Terbium hydride (TbH3) | |
Source | ChemIDplus | |
URL | https://pubchem.ncbi.nlm.nih.gov/substance/?source=chemidplus&sourceid=0013598544 | |
Description | ChemIDplus is a free, web search system that provides access to the structure and nomenclature authority files used for the identification of chemical substances cited in National Library of Medicine (NLM) databases, including the TOXNET system. | |
Record name | Terbium hydride (TbH3) | |
Source | EPA Chemicals under the TSCA | |
URL | https://www.epa.gov/chemicals-under-tsca | |
Description | EPA Chemicals under the Toxic Substances Control Act (TSCA) collection contains information on chemicals and their regulations under TSCA, including non-confidential content from the TSCA Chemical Substance Inventory and Chemical Data Reporting. | |
Record name | Terbium trihydride | |
Source | European Chemicals Agency (ECHA) | |
URL | https://echa.europa.eu/substance-information/-/substanceinfo/100.033.687 | |
Description | The European Chemicals Agency (ECHA) is an agency of the European Union which is the driving force among regulatory authorities in implementing the EU's groundbreaking chemicals legislation for the benefit of human health and the environment as well as for innovation and competitiveness. | |
Explanation | Use of the information, documents and data from the ECHA website is subject to the terms and conditions of this Legal Notice, and subject to other binding limitations provided for under applicable law, the information, documents and data made available on the ECHA website may be reproduced, distributed and/or used, totally or in part, for non-commercial purposes provided that ECHA is acknowledged as the source: "Source: European Chemicals Agency, http://echa.europa.eu/". Such acknowledgement must be included in each copy of the material. ECHA permits and encourages organisations and individuals to create links to the ECHA website under the following cumulative conditions: Links can only be made to webpages that provide a link to the Legal Notice page. | |
Disclaimer and Information on In-Vitro Research Products
Please be aware that all articles and product information presented on BenchChem are intended solely for informational purposes. The products available for purchase on BenchChem are specifically designed for in-vitro studies, which are conducted outside of living organisms. In-vitro studies, derived from the Latin term "in glass," involve experiments performed in controlled laboratory settings using cells or tissues. It is important to note that these products are not categorized as medicines or drugs, and they have not received approval from the FDA for the prevention, treatment, or cure of any medical condition, ailment, or disease. We must emphasize that any form of bodily introduction of these products into humans or animals is strictly prohibited by law. It is essential to adhere to these guidelines to ensure compliance with legal and ethical standards in research and experimentation.