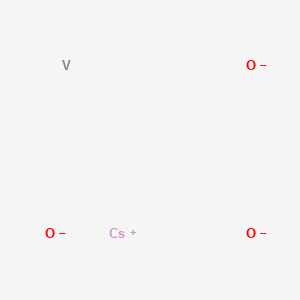
Cesium vanadium oxide (CsVO3)
Overview
Description
Cesium vanadium oxide, with the chemical formula CsVO3, is a compound that combines cesium, vanadium, and oxygen It is known for its unique properties, including its stability and luminescent characteristics
Mechanism of Action
Target of Action
Cesium vanadium oxide (CsVO3) primarily targets optoelectronic applications and biomedical applications . It is also used in perovskite solar cells . The compound’s interaction with these targets is primarily due to its unique optical and electronic properties.
Mode of Action
CsVO3 interacts with its targets through its luminescent properties . It has the highest integrated emission intensity among similar compounds . This makes it particularly useful in optoelectronic applications where light emission is crucial. In perovskite solar cells, CsVO3 acts as the hole extraction layer, enhancing the electrical conductivity of the layer and boosting device performance .
Result of Action
The primary result of CsVO3’s action is the emission of light. It has a high integrated emission intensity, making it useful in applications that require light emission . In perovskite solar cells, the use of CsVO3 results in large fill factors and high short-circuit currents, leading to high power conversion efficiencies .
Action Environment
The action of CsVO3 can be influenced by environmental factors such as temperature. For instance, the compound has been shown to melt congruently in the range of 650–530°C and undergo a reversible phase transition in the range of 520–340°C . These properties could potentially influence its stability and efficacy in different applications.
Preparation Methods
Synthetic Routes and Reaction Conditions: Cesium vanadium oxide can be synthesized through various methods. One common approach is the Pechini process, which involves the reaction of cesium carbonate with vanadium pentoxide in the presence of a chelating agent like citric acid. The mixture is then heated to form a gel, which is subsequently calcined to obtain CsVO3 . Another method involves the evaporation of aqueous solutions of peroxo-isopolyvanadic acid and cesium carbonate, resulting in the formation of CsVO3 nanoparticles .
Industrial Production Methods: In industrial settings, cesium vanadium oxide can be produced using high-temperature solid-state reactions. This involves mixing cesium carbonate and vanadium pentoxide, followed by heating the mixture at elevated temperatures to facilitate the reaction and form the desired compound .
Chemical Reactions Analysis
Types of Reactions: Cesium vanadium oxide undergoes various chemical reactions, including oxidation, reduction, and substitution reactions. For instance, it can participate in redox reactions where vanadium changes its oxidation state.
Common Reagents and Conditions: Typical reagents used in reactions with CsVO3 include reducing agents like hydrogen gas or hydrazine, and oxidizing agents such as oxygen or hydrogen peroxide. These reactions often occur under controlled temperatures and atmospheric conditions to ensure the desired outcomes .
Major Products Formed: The major products formed from reactions involving cesium vanadium oxide depend on the specific reagents and conditions used. For example, reduction reactions may yield lower oxidation state vanadium compounds, while oxidation reactions can produce higher oxidation state vanadium oxides .
Scientific Research Applications
Cesium vanadium oxide has a wide range of scientific research applications:
Optoelectronics: CsVO3 is used in the development of quantum dots for display technologies due to its tunable optical properties.
Catalysis: Its stability at high temperatures makes it a suitable catalyst for various chemical reactions.
Solid-State Electrolytes: CsVO3 is explored as a potential material for solid-state electrolytes in next-generation batteries.
Biomedical Applications: The non-toxic nature of vanadium in its +5 oxidation state makes CsVO3 a candidate for biomedical applications, including imaging and drug delivery.
Comparison with Similar Compounds
- Rubidium vanadium oxide (RbVO3)
- Potassium vanadium oxide (KVO3)
- Sodium vanadium oxide (NaVO3)
Comparison: Cesium vanadium oxide is unique compared to its similar compounds due to its higher stability and luminescent properties. While compounds like rubidium vanadium oxide and potassium vanadium oxide share similar structural characteristics, CsVO3 exhibits superior emission intensity and stability, making it more suitable for applications in optoelectronics and high-temperature catalysis .
Properties
IUPAC Name |
cesium;oxygen(2-);vanadium | |
---|---|---|
Source | PubChem | |
URL | https://pubchem.ncbi.nlm.nih.gov | |
Description | Data deposited in or computed by PubChem | |
InChI |
InChI=1S/Cs.3O.V/q+1;3*-2; | |
Source | PubChem | |
URL | https://pubchem.ncbi.nlm.nih.gov | |
Description | Data deposited in or computed by PubChem | |
InChI Key |
WWENEIGBGOIMMI-UHFFFAOYSA-N | |
Source | PubChem | |
URL | https://pubchem.ncbi.nlm.nih.gov | |
Description | Data deposited in or computed by PubChem | |
Canonical SMILES |
[O-2].[O-2].[O-2].[V].[Cs+] | |
Source | PubChem | |
URL | https://pubchem.ncbi.nlm.nih.gov | |
Description | Data deposited in or computed by PubChem | |
Molecular Formula |
CsO3V-5 | |
Source | PubChem | |
URL | https://pubchem.ncbi.nlm.nih.gov | |
Description | Data deposited in or computed by PubChem | |
DSSTOX Substance ID |
DTXSID50927076 | |
Record name | Cesium vanadium oxide (CsVO3) | |
Source | EPA DSSTox | |
URL | https://comptox.epa.gov/dashboard/DTXSID50927076 | |
Description | DSSTox provides a high quality public chemistry resource for supporting improved predictive toxicology. | |
Molecular Weight |
231.845 g/mol | |
Source | PubChem | |
URL | https://pubchem.ncbi.nlm.nih.gov | |
Description | Data deposited in or computed by PubChem | |
CAS No. |
14644-55-4, 34283-69-7 | |
Record name | Cesium vanadium oxide (CsVO3) | |
Source | ChemIDplus | |
URL | https://pubchem.ncbi.nlm.nih.gov/substance/?source=chemidplus&sourceid=0014644554 | |
Description | ChemIDplus is a free, web search system that provides access to the structure and nomenclature authority files used for the identification of chemical substances cited in National Library of Medicine (NLM) databases, including the TOXNET system. | |
Record name | Cesium vanadium oxide (Cs3VO4) | |
Source | ChemIDplus | |
URL | https://pubchem.ncbi.nlm.nih.gov/substance/?source=chemidplus&sourceid=0034283697 | |
Description | ChemIDplus is a free, web search system that provides access to the structure and nomenclature authority files used for the identification of chemical substances cited in National Library of Medicine (NLM) databases, including the TOXNET system. | |
Record name | Cesium vanadium oxide (CsVO3) | |
Source | EPA Chemicals under the TSCA | |
URL | https://www.epa.gov/chemicals-under-tsca | |
Description | EPA Chemicals under the Toxic Substances Control Act (TSCA) collection contains information on chemicals and their regulations under TSCA, including non-confidential content from the TSCA Chemical Substance Inventory and Chemical Data Reporting. | |
Record name | Cesium vanadium oxide (CsVO3) | |
Source | EPA DSSTox | |
URL | https://comptox.epa.gov/dashboard/DTXSID50927076 | |
Description | DSSTox provides a high quality public chemistry resource for supporting improved predictive toxicology. | |
Record name | Cesium vanadium trioxide | |
Source | European Chemicals Agency (ECHA) | |
URL | https://echa.europa.eu/substance-information/-/substanceinfo/100.035.161 | |
Description | The European Chemicals Agency (ECHA) is an agency of the European Union which is the driving force among regulatory authorities in implementing the EU's groundbreaking chemicals legislation for the benefit of human health and the environment as well as for innovation and competitiveness. | |
Explanation | Use of the information, documents and data from the ECHA website is subject to the terms and conditions of this Legal Notice, and subject to other binding limitations provided for under applicable law, the information, documents and data made available on the ECHA website may be reproduced, distributed and/or used, totally or in part, for non-commercial purposes provided that ECHA is acknowledged as the source: "Source: European Chemicals Agency, http://echa.europa.eu/". Such acknowledgement must be included in each copy of the material. ECHA permits and encourages organisations and individuals to create links to the ECHA website under the following cumulative conditions: Links can only be made to webpages that provide a link to the Legal Notice page. | |
Disclaimer and Information on In-Vitro Research Products
Please be aware that all articles and product information presented on BenchChem are intended solely for informational purposes. The products available for purchase on BenchChem are specifically designed for in-vitro studies, which are conducted outside of living organisms. In-vitro studies, derived from the Latin term "in glass," involve experiments performed in controlled laboratory settings using cells or tissues. It is important to note that these products are not categorized as medicines or drugs, and they have not received approval from the FDA for the prevention, treatment, or cure of any medical condition, ailment, or disease. We must emphasize that any form of bodily introduction of these products into humans or animals is strictly prohibited by law. It is essential to adhere to these guidelines to ensure compliance with legal and ethical standards in research and experimentation.