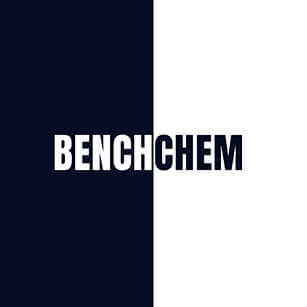
Xenon-124Xe
- Click on QUICK INQUIRY to receive a quote from our team of experts.
- With the quality product at a COMPETITIVE price, you can focus more on your research.
Overview
Description
Xenon-124 (¹²⁴Xe) is a stable isotope of xenon, a noble gas with atomic number 54. It has a natural abundance of 0.0952% in terrestrial xenon reserves and an atomic mass of 123.906 atomic mass units (u) . Unlike radioactive xenon isotopes such as ¹³⁶Xe (half-life > 8.5 × 10²¹ years), ¹²⁴Xe is exceptionally stable, with a half-life exceeding 10¹⁷ years, decaying via double beta (β⁻β⁻) emission . This isotope is commercially available in gaseous form for specialized applications, including nuclear physics research and calibration of particle detectors .
Preparation Methods
Gas Target Technology
The gas target method dominates industrial production due to its compatibility with compact cyclotrons. This approach involves bombarding enriched ¹²⁴Xe gas with protons to generate ¹²³I via intermediate precursors.
Reaction Pathways and Parameters
Two primary nuclear reactions are utilized:
-
¹²⁴Xe(p,2n)¹²³Cs → ¹²³Xe → ¹²³I
-
¹²⁴Xe(p,pn)¹²³Xe → ¹²³I
Key parameters for optimal yield and purity :
The gas is contained in a titanium foil window or cryogenically cooled vessel. The reaction yield depends on stopping power and cross-sections calculated via SRIM simulations .
Challenges and Mitigation Strategies
-
Gas Loss Risk : Accidental rupture of window foils or O-ring failures result in complete gas loss, costing ~$50,000/L. Solutions include redundant seals and rapid evacuation systems .
-
Impurity Management : Xenon-125 (¹²⁵Xe) decay contributes iodine-125 (¹²⁵I) as a contaminant. Purity is optimized by adjusting irradiation/decay times (e.g., <0.2% ¹²⁵I at processing) .
Solid Target Technology
Solid targets address gas loss risks by cryogenically solidifying ¹²⁴Xe. This method reduces xenon consumption and enhances safety.
Design and Operational Parameters
A conical-shaped copper vessel (1 mm thickness, 1 cm outlet diameter) is cooled with liquid nitrogen. Solidified ¹²⁴Xe forms a 0.28 mm layer under 17–28 MeV proton bombardment .
Critical Parameters :
Parameter | Value/Range | Source |
---|---|---|
Target Thickness | 0.28 mm (solidified layer) | |
Beam Current Limit | <1.4 µA (to prevent evaporation) | |
Yield | ~150 mCi/µAh (predicted) |
Advantages Over Gas Targets
Aspect | Gas Target | Solid Target |
---|---|---|
Xenon Usage | 4 g/run | 0.7 g/run (120 cc at STP) |
Safety | High risk of gas loss | Minimal gas leakage risk |
Cost Efficiency | High (gas replacement costs) | Lower (reduced xenon inventory) |
Isotopic Enrichment Techniques
Enriching ¹²⁴Xe is essential for maximizing yield. Gas centrifugation dominates industrial processes.
Gas Centrifugation Process
Orano’s method enriches ¹²⁴Xe to >95% using ultracentrifuges, leveraging xenon’s nine isotopes . Key steps :
-
Feed Gas Preparation : Natural xenon (0.096% ¹²⁴Xe) is purified.
-
Centrifugal Separation : Isotopic masses are separated at high rotational speeds.
-
Product Recovery : Enriched ¹²⁴Xe is cryogenically distilled.
Cost Considerations :
Comparative Analysis of Preparation Methods
Chemical Reactions Analysis
Nuclear Reactions in Astrophysical γ-Process Studies
¹²⁴Xe participates in photodisintegration reactions during the astrophysical γ-process, which synthesizes proton-rich heavy isotopes in stellar environments. Key reactions include:
-
¹²⁴Xe(α,γ)¹²⁸Ba : Radiative alpha capture producing barium-128.
-
¹²⁴Xe(α,n)¹²⁷Ba : Neutron emission channel leading to barium-127.
-
¹²⁴Xe(α,p)¹²⁷Cs : Proton emission channel yielding cesium-127.
Table 1: Decay Parameters of Reaction Products
Isotope | Reaction Channel | Half-Life | Primary γ-Ray Energy (keV) | γ-Ray Intensity (%) |
---|---|---|---|---|
¹²⁸Ba | (α,γ) | 58.32 ± 1.2 h | 273.44 | 14.5 ± 0.7 |
¹²⁷Cs | (α,p) / β⁺-decay | 6.25 ± 0.1 h | 411.95 | 62.9 ± 1.0 |
These cross sections were measured using the activation method, where reaction products are quantified via γ-ray spectroscopy. The ¹²⁴Xe(α,p) channel is complicated by interference from β⁺-decay of ¹²⁷Ba, necessitating precise decay corrections .
Medical Isotope Production via Proton-Induced Reactions
¹²⁴Xe is irradiated with protons to produce iodine-123 (¹²³I), a key diagnostic isotope, through the ¹²⁴Xe(p,2n)¹²³Cs → ¹²³I reaction.
Key Findings :
-
Optimal Energy Range : Proton energies of 25–30 MeV maximize ¹²³I yield while minimizing impurities (e.g., ¹²⁵I).
-
Thick-Target Yield : ~1.2 GBq/µA·h at 27 MeV (after 6.6 hours of bombardment and decay time).
-
Comparative Efficiency : The (p,2n) channel dominates over (p,pn), which has negligible yield (Fig. 2 in ).
Table 2: ¹²⁴Xe Nuclear Reaction Pathways for Medical Isotopes
Target | Reaction | Product | Application |
---|---|---|---|
¹²⁴Xe | (p,2n)¹²³Cs | ¹²³I | Thyroid imaging |
¹²⁴Xe | Neutron capture | ¹²⁵Xe → ¹²⁵I | Cancer therapy |
Experimental Techniques and Challenges
-
Gas Target Systems : High-pressure ¹²⁴Xe gas targets are used in cyclotrons, but require robust containment to prevent gas loss (critical due to ¹²⁴Xe's high cost) .
-
Activation Method : Over 95% of ¹²⁸Ba and ¹²⁷Ba reaction products are captured on foil catchers for analysis, with corrections applied for energy-dependent particle transport simulations .
-
Interference Mitigation : The ¹²⁴Xe(α,p)¹²⁷Cs signal is disentangled from ¹²⁷Ba β⁺-decay using half-life and γ-ray specificity .
Comparative Analysis with Other Xenon Isotopes
While ¹²⁴Xe’s chemical reactivity aligns with natural xenon (e.g., forming XeO₃, XeF₄), its nuclear stability distinguishes it from radioactive isotopes like ¹³³Xe (β⁻-emitter) or ¹³⁵Xe (neutron poison in reactors) .
Scientific Research Applications
Medical Applications
Radiopharmaceutical Precursor
Xenon-124 serves as a precursor for the production of radioactive isotopes such as iodine-123 and iodine-125. These isotopes are crucial in medical diagnostics:
- Iodine-123 : Used for thyroid imaging and diagnosing neurological conditions, including Parkinson's disease.
- Iodine-125 : Utilized in diagnostic imaging for osteoporosis and in radiotherapy for prostate cancer through seed implantation .
Nuclear Physics Research
Double Electron Capture Studies
Xenon-124 is pivotal in nuclear physics research, particularly in studying rare decay processes such as double electron capture. This phenomenon involves the simultaneous capture of two electrons by two protons in the nucleus, transforming them into neutrons while emitting neutrinos. The significance of this process lies in its implications for understanding neutrino properties and nuclear structure:
- The XENON1T experiment at Gran Sasso National Laboratory successfully detected 126 events of double electron capture from Xenon-124, allowing researchers to calculate its half-life to be approximately 1.8×1022 years, which is extraordinarily longer than the age of the universe .
- This detection enhances our understanding of fundamental particles and forces in physics, contributing to the search for dark matter and insights into neutrino behavior .
Astrophysical Applications
Nuclear Reactions in Stars
Xenon-124 plays a role in astrophysical processes, particularly concerning nucleosynthesis in stars:
- Research indicates that reactions involving Xenon-124 can influence the abundance of certain isotopes produced during stellar evolution. For instance, studies on gamma-process reactions highlight how Xenon isotopes contribute to the formation of heavier elements through complex nuclear interactions .
Case Study 1: XENON1T Experiment
The XENON1T experiment is one of the most notable applications of Xenon-124. It aimed to detect dark matter but also provided insights into double electron capture:
- The experiment utilized 3.2 metric tons of liquid xenon to achieve unprecedented sensitivity in detecting rare events. The successful identification of double electron capture events marked a significant milestone in experimental nuclear physics .
Case Study 2: Medical Imaging with Iodine Isotopes
The use of Xenon-124 as a precursor for iodine isotopes showcases its importance in medical diagnostics:
- Clinical studies have demonstrated the effectiveness of iodine-123 in imaging thyroid conditions and assessing neurological diseases, highlighting the direct impact of Xenon-124 on improving patient outcomes through advanced imaging techniques .
Summary Table of Applications
Application Area | Specific Use | Key Findings/Implications |
---|---|---|
Medical Imaging | Precursor for Iodine-123 and Iodine-125 | Enhances diagnostic capabilities for thyroid and neurological conditions |
Nuclear Physics | Double Electron Capture Studies | Provides insights into neutrino properties; half-life measurement aids nuclear structure understanding |
Astrophysics | Influences nucleosynthesis processes | Contributes to understanding element formation in stars |
Mechanism of Action
The primary mechanism of action for xenon-124 involves its radioactive decay through two-neutrino double electron capture. This process occurs when two protons in the xenon nucleus each absorb an electron from the atomic shell and emit a neutrino, converting into neutrons . This decay process is extremely rare and requires highly sensitive detection methods to observe .
Comparison with Similar Compounds
Comparison with Similar Xenon Isotopes
Isotopic Properties and Natural Abundance
The table below compares key properties of ¹²⁴Xe with other stable and long-lived xenon isotopes:
Isotope | Atomic Mass (u) | Natural Abundance (%) | Half-Life | Decay Mode |
---|---|---|---|---|
¹²⁴Xe | 123.906 | 0.0952 | > 10¹⁷ years | β⁻β⁻ |
¹²⁶Xe | 125.904 | 0.089 | Stable | N/A |
¹²⁸Xe | 127.904 | 1.9102 | Stable | N/A |
¹²⁹Xe | 128.905 | 26.4006 | Stable | N/A |
¹³⁶Xe | 135.907 | 8.8573 | > 8.5 × 10²¹ years | β⁻β⁻ |
¹²⁴Xe is distinct due to its low natural abundance (<0.1%) compared to major isotopes like ¹²⁹Xe (26.4%) and ¹³²Xe (26.9%) . Its β⁻β⁻ decay mode is shared only with ¹³⁶Xe, but ¹²⁴Xe’s decay is far less studied due to its extreme stability.
Isotopic Fractionation in Solar System Contexts
Studies of xenon isotopes in the solar system reveal significant isotopic heterogeneity. For example, mixing 22% comet-derived xenon (Comet-Xe) with 78% primordial xenon (Q-Xe) produces a composition close to atmospheric xenon (Start-Xe). However, ¹²⁴Xe in Start-Xe shows a depletion of -162 ± 31‰ relative to solar wind xenon (SW-Xe), far exceeding depletions in ¹²⁶Xe (-77 ± 30‰) and ¹²⁸Xe (-16 ± 37‰) . This suggests ¹²⁴Xe is more sensitive to nucleosynthetic processes or early solar system fractionation mechanisms.
Comparative Analysis of Decay Modes and Stability
¹²⁴Xe and ¹³⁶Xe are the only xenon isotopes confirmed to undergo β⁻β⁻ decay, but their decay rates differ by orders of magnitude. Theoretical models attribute this to differences in nuclear matrix elements and phase-space factors. For instance, ¹³⁶Xe’s shorter half-life (relative to ¹²⁴Xe) aligns with its higher decay energy (Q-value = 2.458 MeV vs. ¹²⁴Xe’s Q = 1.832 MeV) . These differences make ¹²⁴Xe less favorable for current neutrinoless decay experiments but valuable for studying decay mechanisms over cosmological timescales.
Q & A
Basic Research Questions
Q. What experimental methodologies are commonly employed to detect and analyze the decay modes of Xenon-124Xe?
Researchers typically use ultra-low-background detectors like liquid xenon time-projection chambers (LXeTPCs) to study rare decay processes such as two-neutrino double electron capture (2νECEC). These setups minimize cosmic and radiogenic backgrounds through shielding (e.g., water tanks) and material purification . Calibration involves injecting trace amounts of radioactive isotopes (e.g., 83mKr) to validate detector response and energy resolution. Statistical analyses, such as Bayesian inference, are applied to distinguish signal events from noise .
Q. How do researchers design experiments to measure the half-life of this compound’s rare decay processes?
Experimental design focuses on maximizing exposure time and xenon mass while minimizing background interference. For example, the XENON1T experiment utilized 3.2 tonnes of liquid xenon, achieving a half-life sensitivity of 1.1×1022 years for 2νECEC. Key steps include:
- Signal simulation : Monte Carlo methods model expected energy deposition patterns.
- Background reduction : Pulse shape discrimination and fiducial volume cuts isolate decay signals.
- Uncertainty quantification : Systematic errors from detector stability and xenon purity are propagated using covariance matrices .
Q. What role does isotopic enrichment play in this compound studies?
Enrichment of 124Xe (natural abundance: 0.095%) enhances signal yield in rare-event searches. Gas centrifuges or cryogenic distillation are used to achieve >90% isotopic purity. This reduces dilution effects from other xenon isotopes and improves statistical significance in decay measurements .
Advanced Research Questions
Q. How can contradictions in reported half-life values for this compound’s 2νECEC decay be resolved?
Discrepancies often arise from differences in detector calibration, background models, or statistical assumptions. To address these:
- Cross-validation : Compare results across experiments (e.g., XENON1T vs. EXO-200) using standardized data-release protocols.
- Systematic error re-evaluation : Scrutinize energy scale uncertainties and xenon purity thresholds.
- Bayesian model averaging : Combine posterior probabilities from independent analyses to refine consensus values .
Q. What novel statistical frameworks are emerging to improve sensitivity in this compound decay searches?
Machine learning techniques, such as convolutional neural networks (CNNs), are being trained on simulated and experimental data to classify signal vs. background events with higher accuracy. Additionally, frequentist vs. Bayesian hybrid approaches optimize confidence intervals in low-statistics regimes. For example, the XENON collaboration employed a profile likelihood ratio to extract the 2νECEC signal with 3.5σ significance .
Q. How do researchers address the challenge of isotopic interference in multi-xenon systems?
In detectors containing natural xenon, 136Xe (double-beta decay candidate) may dominate backgrounds. Solutions include:
Properties
CAS No. |
15687-60-2 |
---|---|
Molecular Formula |
Xe |
Molecular Weight |
123.90589 g/mol |
IUPAC Name |
xenon-124 |
InChI |
InChI=1S/Xe/i1-7 |
InChI Key |
FHNFHKCVQCLJFQ-NOHWODKXSA-N |
Isomeric SMILES |
[124Xe] |
Canonical SMILES |
[Xe] |
Origin of Product |
United States |
Disclaimer and Information on In-Vitro Research Products
Please be aware that all articles and product information presented on BenchChem are intended solely for informational purposes. The products available for purchase on BenchChem are specifically designed for in-vitro studies, which are conducted outside of living organisms. In-vitro studies, derived from the Latin term "in glass," involve experiments performed in controlled laboratory settings using cells or tissues. It is important to note that these products are not categorized as medicines or drugs, and they have not received approval from the FDA for the prevention, treatment, or cure of any medical condition, ailment, or disease. We must emphasize that any form of bodily introduction of these products into humans or animals is strictly prohibited by law. It is essential to adhere to these guidelines to ensure compliance with legal and ethical standards in research and experimentation.