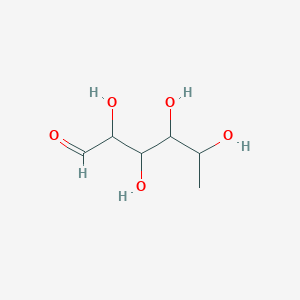
L-(-)-Fucose
Overview
Description
L-(-)-Fucose (C₆H₁₂O₅; CAS 2438-80-4) is a deoxyhexose monosaccharide with a levorotatory configuration, primarily found in glycoproteins, glycolipids, and microbial polysaccharides . It plays critical roles in cell-cell recognition, immune modulation, and microbial interactions. Its structural uniqueness—characterized by a 6-deoxy group and α-(1→2/3/4/6) glycosidic linkages—distinguishes it from other monosaccharides . L-Fucose is biosynthesized via the salvage pathway in mammals, requiring fucokinase (FUK) for phosphorylation , and is industrially produced via engineered Escherichia coli strains or enzymatic isomerization of L-fuculose .
Q & A
Basic Research Questions
Q. What are the standard methodologies for quantifying L-(-)-Fucose in biological samples?
- Methodological Answer : Quantification typically employs high-performance liquid chromatography (HPLC) with refractive index detection or mass spectrometry (GC-MS) for high sensitivity . Enzyme-linked immunosorbent assays (ELISA) using fucose-specific lectins (e.g., Aleuria aurantia lectin) are also common for glycoprotein analysis. For tissue samples, derivatization with trimethylsilyl (TMS) agents enhances volatility in GC-MS workflows .
- Example Workflow:
Extract this compound via methanol-water (80:20) homogenization.
Derivatize with TMS reagents (e.g., BSTFA) for GC-MS analysis.
Validate using internal standards (e.g., deuterated fucose).
Q. How is this compound structurally characterized in glycoconjugates?
- Methodological Answer : Nuclear magnetic resonance (NMR) spectroscopy (¹H/¹³C) identifies anomeric configurations (α/β linkages) and glycosidic bonds . Mass spectrometry (MALDI-TOF or LC-MS/MS) maps fucosylation sites in glycoproteins. For example, α1,2- vs. α1,6-fucosylation can be distinguished via enzymatic digestion with fucosidases .
Q. What experimental models are used to study this compound’s role in microbial adhesion?
- Methodological Answer : In vitro models include:
- Bacterial binding assays (e.g., Helicobacter pylori to gastric epithelial cells) with fucose-coated plates .
- Competitive inhibition using synthetic fucose analogs (e.g., 2'-fucosyllactose) to block lectin-mediated adhesion .
Advanced Research Questions
Q. How can researchers resolve contradictions in reported roles of this compound in inflammatory responses?
- Methodological Answer : Discrepancies (e.g., pro- vs. anti-inflammatory effects in colitis vs. periodontitis ) require:
- Systematic reviews: Meta-analysis of dose-dependent effects across tissues.
- Controlled variables: Standardize animal models (e.g., DSS-induced colitis) and fucose purity (>98% by HPLC ).
- Mechanistic studies: Compare fucose’s impact on TLR4/NF-κB vs. gut microbiota modulation .
Q. What strategies optimize this compound production via microbial fermentation?
- Methodological Answer : Engineered E. coli or Bacillus subtilis strains overexpress fucose synthase (fcs and fkp genes). Key parameters:
- Carbon source: Glycerol > glucose to reduce acetate accumulation .
- Fed-batch fermentation: Maintain microaerobic conditions (DO ~10%) to enhance yield (>50 g/L) .
- Table: Fermentation Optimization Parameters:
Strain | Carbon Source | Yield (g/L) | Reference |
---|---|---|---|
E. coli BL21 | Glycerol | 58.2 | |
B. subtilis | Glucose | 42.7 |
Q. How can this compound’s role in cancer metastasis be mechanistically validated?
- Methodological Answer :
- In vitro: siRNA knockdown of fucosyltransferases (FUT8) in cancer cell lines to assess EMT markers (e.g., E-cadherin loss) .
- In vivo: Xenograft models with fucose-deficient diets to monitor metastatic spread via bioluminescence imaging.
- Multi-omics: Integrate glycomics (LC-MS) and transcriptomics (RNA-seq) to map fucose-dependent pathways .
Q. What methods validate the specificity of this compound in glycan-lectin interactions?
- Methodological Answer : Surface plasmon resonance (SPR) quantifies binding affinity (KD) between fucosylated glycans and lectins (e.g., DC-SIGN). Competitive assays with fucose analogs (e.g., 6-alkynyl fucose) confirm structural specificity .
Q. Data Contradiction Analysis
Q. Why do studies report conflicting α-L-Fucose levels in oral squamous cell carcinoma (OSCC)?
- Methodological Answer : Variability arises from:
- Sample type: Saliva (lower fucose ) vs. tissue biopsies (higher fucose ).
- Detection limits: ELISA vs. mass spectrometry sensitivity .
- Recommendation: Use harmonized protocols (e.g., ISO 15197) for cross-study comparability.
Q. Experimental Design Considerations
Q. How to design a study evaluating this compound’s prebiotic effects?
- Methodological Answer :
- Cohort design: Randomized, double-blind trial with fecal microbiota analysis (16S rRNA sequencing) .
- Controls: Placebo group receiving non-fucosylated oligosaccharides.
- Outcome metrics: Short-chain fatty acid (SCFA) levels via GC-MS.
Q. What in vivo models best recapitulate this compound’s immunomodulatory effects?
Comparison with Similar Compounds
Comparison with Structurally Similar Monosaccharides
L-Rhamnose (6-Deoxy-L-Mannose)
- Structural Differences: L-Rhamnose has a 6-deoxy group like L-fucose but differs in stereochemistry at C2 and C4, making it an epimer of L-fucose. NMR Signature: L-Rhamnose exhibits distinct ¹H-NMR shifts (e.g., 21st chemical shift <96.52 ppm) compared to L-fucose in trisaccharide configurations .
- Functional Contrasts: L-Rhamnose is abundant in plant cell walls and bacterial polysaccharides, whereas L-fucose dominates mammalian glycans and microbial fucoidans .
D-Arabinose
- Isomerization Dynamics: Both L-fucose and D-arabinose are substrates for isomerases (L-FI and D-AI), producing L-fuculose and D-ribulose, respectively. However, L-FI exhibits higher specificity for L-fucose (equilibrium ratio 8.5:1.5 for L-fucose:L-fuculose) .
Comparison in Metabolic Pathways
Microbial Utilization
Mammalian Transport
- GLUT1 vs. Other Transporters: GLUT1 (SLC2A1) is the primary L-fucose transporter in mammals, functioning even at low micromolar concentrations. Macropinocytosis contributes to L-fucose uptake at high concentrations (>25 μM), a mechanism absent in glucose transport .
Functional and Therapeutic Comparisons
Anti-Cancer Biomarkers
Compound | Serum Levels (mg/dl) | Diagnostic Utility |
---|---|---|
L-Fucose | 13.16 (Healthy) | Elevated in dysplasia (22.36 mg/dl) |
Other Glycans | Not specified | Less correlated with dysplasia |
Neuromodulation
- L-Fucose vs. Other Monosaccharides: L-Fucose rescues synaptic plasticity deficits in Alzheimer’s models by upregulating FUK and salvaging glycoprotein degradation . Other monosaccharides (e.g., D-glucose) lack this FUK-dependent neuromodulatory capacity .
Anti-Inflammatory Effects
- Fucoidan (L-Fucose Polymer): Linear fucoidans from Fucus vesiculosus (44% fucose) show superior anti-atherosclerotic activity compared to rhamnose-rich polysaccharides . Sulfation patterns in fucoidan enhance bioactivity, unlike unsulfated L-rhamnose polymers .
Production Methods
Commercial Relevance
- Pharmaceutical Grade L-Fucose: ≥98% purity (HPLC) with applications in monoclonal antibody development and bacterial MCP research . Contrasts with D-arabinose, which lacks direct therapeutic applications .
Preparation Methods
Chemical Synthesis
Acid Hydrolysis of Fucoidan
Fucoidan, a sulfated polysaccharide from brown algae, serves as a primary source for L-fucose via acid hydrolysis. The patented method involves treating fucoidan with hydrochloric acid (HCl) in methanol under pressurized conditions (50–80°C), yielding methyl α-L-fucoside intermediates . Neutralization with lead carbonate removes excess HCl, followed by crystallization (40–50% yield). Subsequent hydrolysis of the fucoside with sulfuric acid produces L-fucose, achieving a total yield of 10–15% from fucoidan . Challenges include lead salt disposal and low overall efficiency due to side reactions.
Chemoenzymatic Conversion from D-Galacturonic Acid
D-Galacturonic acid, derived from pectin hydrolysis, undergoes a four-step transformation to L-fucose :
-
Reduction : Sodium borohydride reduces D-galacturonic acid to L-galactonic acid.
-
Lactonization : Acid-catalyzed cyclization forms L-galactonolactone.
-
Epimerization : Base-mediated inversion at C-3 and C-5 yields L-fuconolactone.
-
Hydrolysis : Acidic hydrolysis of L-fuconolactone produces L-fucose with ~65% overall yield . This route avoids protective group chemistry, enhancing scalability compared to traditional sugar inversions.
Enzymatic Synthesis
L-Fucose Isomerase (L-FucI)-Catalyzed Isomerization
L-FucI enzymes catalyze the reversible isomerization of L-fucose and L-fuculose. Raoultella sp. FucI (RdFucI) favors L-fucose formation, achieving a 9:1 equilibrium ratio at pH 7 and 30°C . Under optimal conditions (40°C, pH 10), RdFucI exhibits a specific activity of 63.9 U/mg for L-fuculose conversion, yielding 90% L-fucose in 12 hours . Structural studies reveal Mn²⁺ dependency and a substrate-binding loop critical for catalytic efficiency .
Chemoenzymatic GDP-L-Fucose Synthesis
The bifunctional enzyme FKP (fucokinase/GDP-fucose pyrophosphorylase) from Bacteroides fragilis enables a two-step pathway:
-
Phosphorylation : L-fucose → L-fucose-1-phosphate (Fuc-1-P).
-
Pyrophosphorylation : Fuc-1-P + GTP → GDP-L-fucose .
Coupled with α1,3-fucosyltransferases, this system synthesizes fucosylated glycans like Lewis X with >80% efficiency .
Microbial Fermentation
Engineered Escherichia coli Strains
Recent metabolic engineering efforts in E. coli have optimized L-fucose production by:
-
Pathway Modularization : Overexpressing GDP-mannose 4,6-dehydratase (GMDS) and GDP-L-fucose synthase (GFUS) to enhance de novo synthesis .
-
Cofactor Recycling : NADPH regeneration via glucose-6-phosphate dehydrogenase overexpression improves GFUS activity .
-
Mixed Carbon Strategy : Co-feeding glucose and glycerol increases ATP availability, achieving 91.90 g/L L-fucose in 5 L bioreactors (1.18 g/L/h productivity) .
Salvage Pathway Utilization
In Helicobacter pylori, the salvage pathway converts free L-fucose to GDP-L-fucose via:
-
Fucokinase (FCSK) : L-fucose → L-fucose-1-phosphate.
-
Fucose-1-phosphate guanylyltransferase (FPGT) : Fuc-1-P + GTP → GDP-L-fucose .
This pathway is less efficient than de novo synthesis but useful in fucose-rich environments .
Extraction from Natural Sources
Algal Hydrolysis
Brown algae (Saccharina japonica, Fucus vesiculosus) contain 5–20% fucoidan by dry weight. Hydrolysis with 2 M HCl at 100°C for 4 hours releases L-fucose, followed by ion-exchange chromatography (Bio-Rad AG 50W-X8) to remove sulfates . Yields range from 8–12%, limited by polysaccharide heterogeneity .
Comparative Analysis of Methods
Method | Substrate | Yield | Time | Cost | Scalability |
---|---|---|---|---|---|
Acid Hydrolysis | Fucoidan | 10–15% | 24–48 h | Low | Moderate |
Chemoenzymatic (D-GalA) | D-Galacturonic acid | 65% | 72 h | Medium | High |
E. coli Fermentation | Glucose/Glycerol | 91.90 g/L | 78 h | High | Industrial |
Enzymatic Isomerization | L-Fuculose | 90% | 12 h | Medium | Pilot-scale |
Key Findings :
-
Microbial fermentation surpasses chemical methods in yield (91.90 g/L vs. <20%) but requires advanced bioreactor setups .
-
Enzymatic isomerization offers high specificity but depends on costly L-fuculose substrates .
-
Algal extraction is environmentally sustainable but hindered by low yields and purification challenges .
Properties
CAS No. |
75247-25-5 |
---|---|
Molecular Formula |
C6H12O5 |
Molecular Weight |
164.16 g/mol |
IUPAC Name |
2,3,4,5-tetrahydroxyhexanal |
InChI |
InChI=1S/C6H12O5/c1-3(8)5(10)6(11)4(9)2-7/h2-6,8-11H,1H3 |
InChI Key |
PNNNRSAQSRJVSB-UHFFFAOYSA-N |
Canonical SMILES |
CC(C(C(C(C=O)O)O)O)O |
Origin of Product |
United States |
Synthesis routes and methods
Procedure details
Retrosynthesis Analysis
AI-Powered Synthesis Planning: Our tool employs the Template_relevance Pistachio, Template_relevance Bkms_metabolic, Template_relevance Pistachio_ringbreaker, Template_relevance Reaxys, Template_relevance Reaxys_biocatalysis model, leveraging a vast database of chemical reactions to predict feasible synthetic routes.
One-Step Synthesis Focus: Specifically designed for one-step synthesis, it provides concise and direct routes for your target compounds, streamlining the synthesis process.
Accurate Predictions: Utilizing the extensive PISTACHIO, BKMS_METABOLIC, PISTACHIO_RINGBREAKER, REAXYS, REAXYS_BIOCATALYSIS database, our tool offers high-accuracy predictions, reflecting the latest in chemical research and data.
Strategy Settings
Precursor scoring | Relevance Heuristic |
---|---|
Min. plausibility | 0.01 |
Model | Template_relevance |
Template Set | Pistachio/Bkms_metabolic/Pistachio_ringbreaker/Reaxys/Reaxys_biocatalysis |
Top-N result to add to graph | 6 |
Feasible Synthetic Routes
Disclaimer and Information on In-Vitro Research Products
Please be aware that all articles and product information presented on BenchChem are intended solely for informational purposes. The products available for purchase on BenchChem are specifically designed for in-vitro studies, which are conducted outside of living organisms. In-vitro studies, derived from the Latin term "in glass," involve experiments performed in controlled laboratory settings using cells or tissues. It is important to note that these products are not categorized as medicines or drugs, and they have not received approval from the FDA for the prevention, treatment, or cure of any medical condition, ailment, or disease. We must emphasize that any form of bodily introduction of these products into humans or animals is strictly prohibited by law. It is essential to adhere to these guidelines to ensure compliance with legal and ethical standards in research and experimentation.