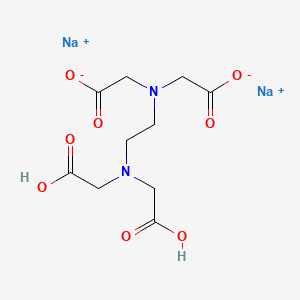
EDTA disodium salt
Overview
Description
Ethylenediaminetetraacetic acid disodium salt (EDTA disodium salt) is a chelating agent with the chemical formula C₁₀H₁₄N₂Na₂O₈ and molecular weight 336.21 g/mol (anhydrous) or 372.24 g/mol (dihydrate) . It exists as a white crystalline powder, highly soluble in water, and forms stable complexes with divalent and trivalent metal ions (e.g., Ca²⁺, Mg²⁺, Fe³⁺) . Its applications span pharmaceuticals (e.g., anticoagulants, metal poisoning treatment), industrial processes (nanomaterial synthesis, corrosion inhibition), and environmental remediation (heavy metal removal) .
Preparation Methods
Synthetic Routes and Reaction Conditions
EDTA disodium salt can be synthesized by reacting ethylenediamine with formaldehyde and sodium cyanide, followed by hydrolysis. The reaction conditions typically involve an alkaline medium to facilitate the formation of the disodium salt .
Industrial Production Methods
In industrial settings, disodium ethylenediaminetetraacetate is produced by dissolving ethylenediaminetetraacetic acid in sodium hydroxide solution. The mixture is then heated to promote the formation of the disodium salt. The resulting solution is filtered and crystallized to obtain pure disodium ethylenediaminetetraacetate .
Chemical Reactions Analysis
Metal Complexation Reactions
EDTA disodium salt acts as a hexadentate ligand, binding metal ions via two amine and four carboxylate groups. Key reactions include:
General Complexation Equation
Examples :
-
Calcium :
-
Iron(III) :
Equilibrium Constants for Select Metal Complexes
Metal Ion | Log Stability Constant () | pH Range for Stability |
---|---|---|
Ca²⁺ | 10.7 | 8–12 |
Mg²⁺ | 8.7 | 9–11 |
Fe³⁺ | 25.1 | 1–3 |
Cu²⁺ | 18.8 | 3–5 |
Zn²⁺ | 16.5 | 4–6 |
Data compiled from . |
pH-Dependent Reactivity
The chelating efficiency of this compound varies with pH due to protonation equilibria:
-
Below pH 2 : Carboxyl groups are protonated, reducing ligand availability .
-
pH 6–12 : Optimal deprotonation enables effective binding to divalent (Ca²⁺, Mg²⁺) and trivalent (Fe³⁺) ions .
Protonation Scheme :
pKₐ values: 0, 1.5, 2.0, 2.66 (carboxyl), 6.16, 10.24 (amine) .
Water Softening
This compound reacts with Ca²⁺/Mg²⁺ in hard water, forming soluble complexes to prevent scale formation :
Titrimetric Analysis
Used in EDTA titrations for metal quantification (e.g., water hardness testing) :
-
Endpoint Detection : Eriochrome Black T indicator transitions from wine-red (metal-bound) to blue (EDTA-bound) .
Reactivity with Organic Compounds
This compound enhances solubility of hydrophobic metal complexes, enabling applications in:
-
Photography : Prevents fogging by sequestering Cu²⁺/Fe³⁺ in developers .
-
Textile Dyeing : Stabilizes dye-metal complexes for color retention .
Toxicological Interactions
-
Metal Deficiency : Prolonged exposure depletes Zn²⁺, Ca²⁺, and Fe³⁺, leading to systemic toxicity .
-
Ecotoxicity : Disrupts metal adsorption in wastewater treatment, increasing heavy metal bioavailability .
This compound’s versatility stems from its robust chelation chemistry, pH sensitivity, and stability across industrial and biological systems. Its reactions are foundational to applications ranging from analytical chemistry to environmental management, though its use requires careful consideration of ecological and health impacts .
Scientific Research Applications
EDTA disodium salt has a wide range of scientific research applications:
Chemistry: It is used as a chelating agent in complexometric titrations to determine metal ion concentrations.
Biology: It inhibits metalloproteases and other enzymes that require metal ions for activity.
Medicine: It is used in chelation therapy to treat heavy metal poisoning, such as lead and mercury toxicity.
Mechanism of Action
EDTA disodium salt exerts its effects by chelating metal ions. It forms an octahedral complex with divalent cations, effectively sequestering them and preventing their participation in biochemical reactions. This mechanism is particularly useful in inhibiting enzymes that require metal ions for their activity .
Comparison with Similar Compounds
EDTA Disodium Salt vs. Calcium Disodium EDTA
Key Insight : this compound should never substitute calcium disodium EDTA in medical treatments, as improper use can cause life-threatening hypocalcemia, seizures, or cardiac arrest .
This compound vs. EDTA Tetrasodium Salt
Key Insight : The tetrasodium salt’s higher pH and chelation capacity make it superior in industrial processes requiring alkaline conditions .
This compound vs. Free EDTA Acid
Key Insight : The disodium salt’s superior solubility and pH stability make it a default choice in consumer products .
This compound vs. Alternative Chelators
Citric Acid (CIT) and Nitrilotriacetic Acid (NTA)
- Efficacy in Heavy Metal Removal :
HEDPA (Hydroxyethylidene Diphosphonic Acid)
- Biofilm Removal : EDTA disodium shows moderate efficacy (~50% biofilm removal) but synergizes with lysozyme at low concentrations (0.5–50 g/L) .
Q & A
Basic Research Questions
Q. How should EDTA disodium salt standard solutions be prepared for titrimetric analysis in metal quantification?
this compound is commonly used in complexometric titrations due to its high affinity for divalent and trivalent cations. To prepare a 0.05 M standard solution:
- Dissolve 18.61 g of this compound dihydrate (372.24 g/mol) in 1 L of deionized water. Adjust pH to 8.0–10.0 using NaOH to ensure solubility and stability .
- For calcium determination, ash the sample, dilute in water, and alkalize to pH 12.5–13 to precipitate magnesium as Mg(OH)₂, allowing selective Ca²⁺ titration with EDTA using calcon carboxylic acid as an indicator .
Q. What precautions are necessary for handling and storing this compound in laboratory settings?
- Storage: Keep in a tightly sealed container at room temperature (15–25°C), away from moisture and incompatible materials (e.g., strong oxidizers, aluminum) .
- Safety: Use PPE (gloves, goggles) to avoid eye irritation (classified as Hazard Category 2B) and respiratory exposure. Although non-flammable, avoid dust generation .
Q. How does pH influence the chelation efficiency of this compound in aqueous solutions?
this compound exhibits optimal metal-binding capacity at pH 8–10. Below pH 4, protonation reduces its ability to form stable complexes. For example, in acidic conditions (pH < 6), EDTA may precipitate, requiring pH adjustment with NaOH to maintain solubility .
Advanced Research Questions
Q. How can researchers optimize this compound in factorial experimental designs for material synthesis?
A 3² factorial design was used to optimize chitosan–EDTA microparticles, varying the EDTA disodium/chitosan ratio (1:1 to 1:3) and processing temperature (25–45°C). Key outputs included particle size (0.5–2.0 µm) and zeta potential (−25 to −40 mV), with higher EDTA ratios enhancing crosslinking but requiring dropwise addition to prevent precipitation .
Q. How to reconcile contradictory ecotoxicological data for this compound in environmental studies?
- Aquatic toxicity: Reported LC50 values vary (e.g., 1.1–7.6 mg/L for bluegill sunfish, 5.0 mg/L for Daphnia), likely due to differences in test conditions (pH, water hardness). EDTA’s low log Kow (1.48) and BCF (<10) suggest minimal bioaccumulation, but its persistence in water warrants caution .
- Regulatory discrepancies: Some SDS classify it as non-hazardous, while others note eye irritation (H319) and aquatic toxicity (H402). Researchers must consult regional guidelines (e.g., EPA, REACH) for hazard classification .
Q. What analytical methods are recommended for detecting this compound in complex matrices (e.g., biological samples)?
- GC/MS-SIM: Derivatize EDTA in water or tissue samples using BF₃-methanol for methylation, followed by GC/MS-SIM with a detection limit of 0.2–10 µg/L. Internal standards (e.g., EDTA-d12) improve accuracy in low-recovery scenarios (e.g., 60% recovery in sediment) .
- HPLC: Pair with UV detection (254 nm) for pharmaceutical-grade purity testing (99.0–101.0% per USP/Ph. Eur.) .
Q. How does this compound interact with microbial communities in environmental or bioreactor studies?
In enhanced biological phosphorus removal (EBPR) systems, EDTA (0.1 g/L) is added to synthetic media to chelate trace metals (Fe³⁺, Cu²⁺), preventing enzyme inhibition. However, excess EDTA may disrupt metal-dependent metabolic pathways, requiring dose optimization via batch testing .
Q. What factors influence the stability of this compound in long-term experiments?
- Thermal stability: Solutions can be autoclaved (121°C, 15 psi) without degradation, but prolonged heating in acidic conditions (>pH 5) may hydrolyze the EDTA–metal complex .
- Light exposure: UV absorption at 300–380 nm is weak (extinction coefficient <30/M·cm), suggesting limited photodegradation under standard lab conditions .
Q. Methodological Considerations
Q. How to validate this compound’s role in mitigating matrix interference during ICP-MS analysis?
- Spike recovery tests: Add known EDTA concentrations to samples containing interfering ions (e.g., Ca²⁺, Fe³⁺). Recovery rates <90% indicate insufficient chelation, requiring pH adjustment or higher EDTA doses .
- Blank correction: Account for EDTA’s background signal by analyzing metal-free EDTA solutions .
Q. What statistical approaches address variability in EDTA-dependent experimental outcomes?
- Use ANOVA to assess the significance of factors like pH, temperature, and EDTA concentration. For example, a 95% confidence interval confirmed that EDTA/chitosan ratio (p < 0.01) had a greater impact on microparticle size than temperature .
- Bootstrap resampling is recommended for small datasets (n < 30) to estimate confidence intervals for LC50 values .
Properties
Molecular Formula |
C10H14N2Na2O8 |
---|---|
Molecular Weight |
336.21 g/mol |
IUPAC Name |
disodium;2-[2-[bis(carboxymethyl)amino]ethyl-(carboxylatomethyl)amino]acetate |
InChI |
InChI=1S/C10H16N2O8.2Na/c13-7(14)3-11(4-8(15)16)1-2-12(5-9(17)18)6-10(19)20;;/h1-6H2,(H,13,14)(H,15,16)(H,17,18)(H,19,20);;/q;2*+1/p-2 |
InChI Key |
ZGTMUACCHSMWAC-UHFFFAOYSA-L |
Canonical SMILES |
C(CN(CC(=O)[O-])CC(=O)[O-])N(CC(=O)O)CC(=O)O.[Na+].[Na+] |
Origin of Product |
United States |
Synthesis routes and methods
Procedure details
Retrosynthesis Analysis
AI-Powered Synthesis Planning: Our tool employs the Template_relevance Pistachio, Template_relevance Bkms_metabolic, Template_relevance Pistachio_ringbreaker, Template_relevance Reaxys, Template_relevance Reaxys_biocatalysis model, leveraging a vast database of chemical reactions to predict feasible synthetic routes.
One-Step Synthesis Focus: Specifically designed for one-step synthesis, it provides concise and direct routes for your target compounds, streamlining the synthesis process.
Accurate Predictions: Utilizing the extensive PISTACHIO, BKMS_METABOLIC, PISTACHIO_RINGBREAKER, REAXYS, REAXYS_BIOCATALYSIS database, our tool offers high-accuracy predictions, reflecting the latest in chemical research and data.
Strategy Settings
Precursor scoring | Relevance Heuristic |
---|---|
Min. plausibility | 0.01 |
Model | Template_relevance |
Template Set | Pistachio/Bkms_metabolic/Pistachio_ringbreaker/Reaxys/Reaxys_biocatalysis |
Top-N result to add to graph | 6 |
Feasible Synthetic Routes
Disclaimer and Information on In-Vitro Research Products
Please be aware that all articles and product information presented on BenchChem are intended solely for informational purposes. The products available for purchase on BenchChem are specifically designed for in-vitro studies, which are conducted outside of living organisms. In-vitro studies, derived from the Latin term "in glass," involve experiments performed in controlled laboratory settings using cells or tissues. It is important to note that these products are not categorized as medicines or drugs, and they have not received approval from the FDA for the prevention, treatment, or cure of any medical condition, ailment, or disease. We must emphasize that any form of bodily introduction of these products into humans or animals is strictly prohibited by law. It is essential to adhere to these guidelines to ensure compliance with legal and ethical standards in research and experimentation.