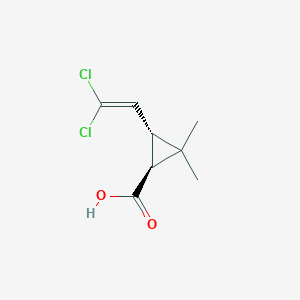
1S-trans-Permethrinic acid
Overview
Description
1S-trans-Permethrinic acid is a chemical compound with the molecular formula C8H10Cl2O2. It is an intermediate in the synthesis of trans-Permethrin, which is a common synthetic pyrethroid insecticide known for its low mammalian toxicity . The compound is characterized by its structure, which includes a cyclopropanecarboxylic acid moiety with dichlorovinyl and dimethyl substituents.
Preparation Methods
Synthetic Routes and Reaction Conditions
1S-trans-Permethrinic acid can be synthesized through a variation of the conventional chrysanthemic acid synthesis. This involves the reaction of 1,1-dichloro-4-methyl-1,3-pentadiene with ethyldiazoacetate in the presence of a copper catalyst . The resulting product undergoes further processing to yield this compound.
Industrial Production Methods
Industrial production of this compound typically involves large-scale synthesis using optimized reaction conditions to ensure high yield and purity. The process may include steps such as distillation, crystallization, and purification to obtain the final product suitable for use as an intermediate in insecticide production.
Chemical Reactions Analysis
Types of Reactions
1S-trans-Permethrinic acid undergoes various chemical reactions, including:
Oxidation: The compound can be oxidized to form corresponding carboxylic acids or other oxidized derivatives.
Reduction: Reduction reactions can convert the compound into alcohols or other reduced forms.
Substitution: The dichlorovinyl group can participate in substitution reactions, leading to the formation of different derivatives.
Common Reagents and Conditions
Oxidation: Common oxidizing agents include potassium permanganate and chromium trioxide.
Reduction: Reducing agents such as lithium aluminum hydride or sodium borohydride are used.
Substitution: Reagents like sodium hydroxide or other nucleophiles can facilitate substitution reactions.
Major Products Formed
The major products formed from these reactions depend on the specific conditions and reagents used. For example, oxidation may yield carboxylic acids, while reduction can produce alcohols.
Scientific Research Applications
1S-trans-Permethrinic acid is primarily used as an intermediate in the synthesis of trans-Permethrin, a widely used insecticide. Its applications extend to various fields:
Chemistry: Used in the synthesis of other pyrethroid compounds.
Biology: Studied for its effects on insect physiology and behavior.
Medicine: Investigated for potential use in treating parasitic infections.
Industry: Employed in the production of insecticides for agricultural and domestic use.
Mechanism of Action
The mechanism of action of 1S-trans-Permethrinic acid, as an intermediate in trans-Permethrin synthesis, involves its conversion to the active insecticidal form. Trans-Permethrin acts on the nerve cell membrane of insects, disrupting the sodium channel current and causing delayed repolarization and paralysis . This disruption leads to the effective control of insect pests.
Comparison with Similar Compounds
1S-trans-Permethrinic acid is unique due to its specific configuration and role as an intermediate in pyrethroid synthesis. Similar compounds include:
Permethrin: The parent compound, used as an insecticide.
Cypermethrin: Another pyrethroid with similar insecticidal properties.
Cyfluthrin: A pyrethroid with a fluorine substitution, exhibiting different biological activity and toxicity.
These compounds share structural similarities but differ in their specific substituents and biological activities, making this compound a valuable intermediate in the synthesis of effective insecticides.
Properties
Molecular Formula |
C8H10Cl2O2 |
---|---|
Molecular Weight |
209.07 g/mol |
IUPAC Name |
(1S,3R)-3-(2,2-dichloroethenyl)-2,2-dimethylcyclopropane-1-carboxylic acid |
InChI |
InChI=1S/C8H10Cl2O2/c1-8(2)4(3-5(9)10)6(8)7(11)12/h3-4,6H,1-2H3,(H,11,12)/t4-,6+/m0/s1 |
InChI Key |
LLMLSUSAKZVFOA-UJURSFKZSA-N |
Isomeric SMILES |
CC1([C@H]([C@@H]1C(=O)O)C=C(Cl)Cl)C |
Canonical SMILES |
CC1(C(C1C(=O)O)C=C(Cl)Cl)C |
Origin of Product |
United States |
Synthesis routes and methods I
Procedure details
Synthesis routes and methods II
Procedure details
Synthesis routes and methods III
Procedure details
Retrosynthesis Analysis
AI-Powered Synthesis Planning: Our tool employs the Template_relevance Pistachio, Template_relevance Bkms_metabolic, Template_relevance Pistachio_ringbreaker, Template_relevance Reaxys, Template_relevance Reaxys_biocatalysis model, leveraging a vast database of chemical reactions to predict feasible synthetic routes.
One-Step Synthesis Focus: Specifically designed for one-step synthesis, it provides concise and direct routes for your target compounds, streamlining the synthesis process.
Accurate Predictions: Utilizing the extensive PISTACHIO, BKMS_METABOLIC, PISTACHIO_RINGBREAKER, REAXYS, REAXYS_BIOCATALYSIS database, our tool offers high-accuracy predictions, reflecting the latest in chemical research and data.
Strategy Settings
Precursor scoring | Relevance Heuristic |
---|---|
Min. plausibility | 0.01 |
Model | Template_relevance |
Template Set | Pistachio/Bkms_metabolic/Pistachio_ringbreaker/Reaxys/Reaxys_biocatalysis |
Top-N result to add to graph | 6 |
Feasible Synthetic Routes
Disclaimer and Information on In-Vitro Research Products
Please be aware that all articles and product information presented on BenchChem are intended solely for informational purposes. The products available for purchase on BenchChem are specifically designed for in-vitro studies, which are conducted outside of living organisms. In-vitro studies, derived from the Latin term "in glass," involve experiments performed in controlled laboratory settings using cells or tissues. It is important to note that these products are not categorized as medicines or drugs, and they have not received approval from the FDA for the prevention, treatment, or cure of any medical condition, ailment, or disease. We must emphasize that any form of bodily introduction of these products into humans or animals is strictly prohibited by law. It is essential to adhere to these guidelines to ensure compliance with legal and ethical standards in research and experimentation.