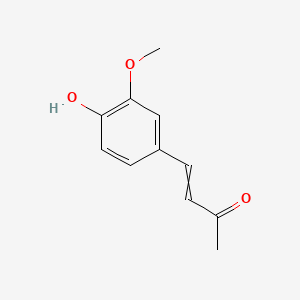
Vanillylidene acetone
Overview
Description
Vanillylidene acetone, also known as dehydrozingerone or vanillylideneacetone, is an organic compound with the molecular formula C11H12O3 and a molecular weight of 192.2112 g/mol . This compound is a derivative of zingerone, a phenolic compound found in ginger. It is characterized by the presence of a butenone group conjugated to a hydroxy-methoxyphenyl group, which imparts unique chemical and biological properties.
Preparation Methods
Synthetic Routes and Reaction Conditions
The synthesis of Vanillylidene acetone can be achieved through various methods. One common synthetic route involves the condensation of vanillin (4-hydroxy-3-methoxybenzaldehyde) with acetone in the presence of a base such as sodium hydroxide. The reaction proceeds via an aldol condensation mechanism, followed by dehydration to yield the desired product .
Industrial Production Methods
Industrial production of this compound typically involves large-scale aldol condensation reactions. The reaction conditions are optimized to ensure high yield and purity of the product. The use of catalysts and controlled reaction environments are common practices to enhance the efficiency of the synthesis process .
Chemical Reactions Analysis
Types of Reactions
Vanillylidene acetone undergoes various chemical reactions, including:
Oxidation: The compound can be oxidized to form corresponding carboxylic acids or quinones.
Reduction: Reduction reactions can convert the butenone group to a butanol group.
Substitution: The phenolic hydroxyl group can undergo electrophilic substitution reactions, such as nitration or halogenation.
Common Reagents and Conditions
Oxidation: Common oxidizing agents include potassium permanganate (KMnO4) and chromium trioxide (CrO3).
Reduction: Reducing agents such as sodium borohydride (NaBH4) or lithium aluminum hydride (LiAlH4) are used.
Substitution: Electrophilic substitution reactions often require acidic conditions and reagents like nitric acid (HNO3) or bromine (Br2).
Major Products Formed
Oxidation: Formation of carboxylic acids or quinones.
Reduction: Formation of butanol derivatives.
Substitution: Formation of nitro or halogenated derivatives.
Scientific Research Applications
Vanillylidene acetone has diverse applications in scientific research:
Chemistry: Used as a building block in organic synthesis and as a precursor for the synthesis of more complex molecules.
Biology: Studied for its antioxidant and anti-inflammatory properties.
Medicine: Investigated for potential therapeutic effects, including anticancer and antimicrobial activities.
Industry: Utilized in the production of flavoring agents and fragrances
Mechanism of Action
The biological effects of Vanillylidene acetone are attributed to its ability to interact with various molecular targets and pathways. The compound can modulate oxidative stress by scavenging free radicals and enhancing antioxidant enzyme activities. It also influences inflammatory pathways by inhibiting the production of pro-inflammatory cytokines and enzymes .
Comparison with Similar Compounds
Similar Compounds
Zingerone: 4-(4-hydroxy-3-methoxyphenyl)-2-butanone, a closely related compound found in ginger.
Vanillin: 4-hydroxy-3-methoxybenzaldehyde, a precursor in the synthesis of Vanillylidene acetone.
Eugenol: 4-allyl-2-methoxyphenol, another phenolic compound with similar structural features
Uniqueness
This compound is unique due to its conjugated butenone structure, which imparts distinct chemical reactivity and biological activity compared to its analogs. Its ability to undergo various chemical transformations and its potential therapeutic applications make it a valuable compound in research and industry .
Properties
Molecular Formula |
C11H12O3 |
---|---|
Molecular Weight |
192.21 g/mol |
IUPAC Name |
4-(4-hydroxy-3-methoxyphenyl)but-3-en-2-one |
InChI |
InChI=1S/C11H12O3/c1-8(12)3-4-9-5-6-10(13)11(7-9)14-2/h3-7,13H,1-2H3 |
InChI Key |
AFWKBSMFXWNGRE-UHFFFAOYSA-N |
Canonical SMILES |
CC(=O)C=CC1=CC(=C(C=C1)O)OC |
Origin of Product |
United States |
Synthesis routes and methods I
Procedure details
Synthesis routes and methods II
Procedure details
Retrosynthesis Analysis
AI-Powered Synthesis Planning: Our tool employs the Template_relevance Pistachio, Template_relevance Bkms_metabolic, Template_relevance Pistachio_ringbreaker, Template_relevance Reaxys, Template_relevance Reaxys_biocatalysis model, leveraging a vast database of chemical reactions to predict feasible synthetic routes.
One-Step Synthesis Focus: Specifically designed for one-step synthesis, it provides concise and direct routes for your target compounds, streamlining the synthesis process.
Accurate Predictions: Utilizing the extensive PISTACHIO, BKMS_METABOLIC, PISTACHIO_RINGBREAKER, REAXYS, REAXYS_BIOCATALYSIS database, our tool offers high-accuracy predictions, reflecting the latest in chemical research and data.
Strategy Settings
Precursor scoring | Relevance Heuristic |
---|---|
Min. plausibility | 0.01 |
Model | Template_relevance |
Template Set | Pistachio/Bkms_metabolic/Pistachio_ringbreaker/Reaxys/Reaxys_biocatalysis |
Top-N result to add to graph | 6 |
Feasible Synthetic Routes
Disclaimer and Information on In-Vitro Research Products
Please be aware that all articles and product information presented on BenchChem are intended solely for informational purposes. The products available for purchase on BenchChem are specifically designed for in-vitro studies, which are conducted outside of living organisms. In-vitro studies, derived from the Latin term "in glass," involve experiments performed in controlled laboratory settings using cells or tissues. It is important to note that these products are not categorized as medicines or drugs, and they have not received approval from the FDA for the prevention, treatment, or cure of any medical condition, ailment, or disease. We must emphasize that any form of bodily introduction of these products into humans or animals is strictly prohibited by law. It is essential to adhere to these guidelines to ensure compliance with legal and ethical standards in research and experimentation.