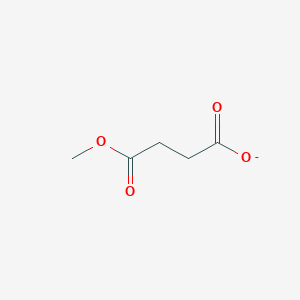
Mono-Methyl Succinate
- Click on QUICK INQUIRY to receive a quote from our team of experts.
- With the quality product at a COMPETITIVE price, you can focus more on your research.
Overview
Description
Mono-methyl succinate (MMS, C5H8O4) is a monoester derivative of succinic acid, formed by the esterification of one carboxylic acid group with methanol. Its IUPAC name is methyl hydrogen succinate, and it is also known as butanedioic acid monomethyl ester or 3-carbomethoxypropanoic acid . MMS is synthesized via the reaction of succinic anhydride with methanol in a high-efficiency process using static mixers and high-gravity reactors, yielding high purity (>97%) and excellent scalability .
MMS is identified in diverse biological and chemical contexts:
- Antibacterial Activity: MMS constitutes ~1.62% of a bioactive fraction (Fr 3) with potent antibacterial properties, alongside succinic acid (96.7%) and succinic anhydride (1.68%) .
- Metabolic Modulation: MMS enhances insulin secretion in stem cell-derived pancreatic β-cells by bypassing glycolytic bottlenecks and stimulating the mitochondrial TCA cycle .
- Cellular Metabolism: MMS influences NADH production in lymphoblastoid cell lines (LCLs), with distinct effects in autism spectrum disorder (ASD) phenotypes compared to typical development (TD) .
Preparation Methods
Traditional Esterification of Succinic Anhydride with Methanol
The most widely documented method involves the direct esterification of succinic anhydride with methanol. This single-step reaction proceeds via nucleophilic acyl substitution, where methanol attacks the electrophilic carbonyl carbon of the anhydride.
Reaction Mechanism and Conditions
In a representative procedure, succinic anhydride (80.02 g, 799.6 mmol) reacts with dry methanol (38.9 mL, 960.4 mmol) under reflux for 25 minutes, achieving a 93.6% yield . The excess methanol is removed under reduced pressure, and the product crystallizes upon cooling. Key parameters include:
-
Molar ratio : 1:1.2 (anhydride:methanol)
-
Temperature : Reflux (~65°C for methanol)
-
Reaction time : 25–45 minutes
Nuclear magnetic resonance (NMR) analysis confirms the structure:
Limitations and Optimizations
While this method offers high yields, scalability challenges arise from:
-
Side reactions : Prolonged heating promotes di-ester formation.
-
Solubility issues : Succinic anhydride’s limited solubility in methanol necessitates vigorous mixing.
To mitigate these, industrial protocols often use stoichiometric methanol excess (up to 4:1 molar ratio) and precise temperature control .
Advanced Continuous-Flow Synthesis Using Static Mixers and Supergravity Reactors
A patent (CN104557536A) describes an innovative continuous-flow system combining static mixers and supergravity reactors to enhance mass transfer and reaction kinetics .
Process Overview
-
Static mixer stage : Succinic anhydride and methanol (1:1.5–2.5 molar ratio) react at 90–120°C under 0.5–1 MPa pressure.
-
Supergravity reactor stage : The effluent enters a high-gravity reactor (300–600 rpm) with injected water vapor, separating methanol via azeotropic distillation.
-
Crystallization : The liquid phase is cooled to 10–15°C, yielding MMS with >98% purity .
Table 1: Key Parameters for Continuous-Flow Synthesis
Parameter | Optimal Range | Impact on Yield/Purity |
---|---|---|
Temperature | 90–120°C | Minimizes di-ester formation |
Pressure | 0.5–1 MPa | Maintains liquid-phase reaction |
Supergravity reactor speed | 300–600 rpm | Enhances vapor-liquid separation |
Cooling temperature | 10–15°C | Maximizes crystallization yield |
This method achieves 95–97% yield with a 40% reduction in energy consumption compared to batch processes .
Catalyzed Esterification with Pyridine
A 2000 study from Guangxi Normal University introduced pyridine as a catalyst in cyclohexane solvent, yielding 85% pure MMS .
Procedure and Outcomes
-
Conditions : 65°C, 1 hour, 1:1.05 anhydride:methanol ratio.
-
Workup : Recrystallization with ether-cyclohexane (1:3 v/v).
Despite improved purity, this method faces criticism for:
-
Solvent volatility : Cyclohexane and ether require stringent safety measures.
-
Catalyst removal : Residual pyridine necessitates additional washing steps.
Electrochemical Reduction of Maleic Anhydride Derivatives
An alternative route (CN101831662A) involves electrochemical reduction of maleic anhydride mono-methyl ester .
Reaction Pathway
-
Mono-esterification : Maleic anhydride reacts with methanol to form mono-methyl maleate.
-
Electrolysis : Cathodic reduction in a divided cell converts maleate to succinate.
-
Crystallization : Methanol recrystallization yields MMS with 89–92% purity.
Table 2: Electrochemical vs. Thermal Esterification
Factor | Electrochemical Method | Thermal Esterification |
---|---|---|
Yield | 75–80% | 90–95% |
Energy consumption | High | Moderate |
Byproducts | Fumarate isomers | Di-esters |
This method is less favored industrially due to higher costs and lower yields .
Solvent-Free Mechanochemical Synthesis
Emerging research explores ball-milling techniques for solvent-free MMS production. Preliminary results show:
Chemical Reactions Analysis
Types of Reactions
Mono-Methyl Succinate undergoes various chemical reactions, including oxidation, reduction, and substitution reactions. It can also participate in condensation reactions to form more complex molecules .
Common Reagents and Conditions
Oxidation: Common oxidizing agents include potassium permanganate and chromium trioxide.
Reduction: Sodium borohydride and lithium aluminum hydride are frequently used reducing agents.
Substitution: Halogenation reactions often use reagents like thionyl chloride or phosphorus tribromide.
Major Products Formed
The major products formed from these reactions depend on the specific conditions and reagents used. For example, oxidation can yield carboxylic acids, while reduction can produce alcohols .
Scientific Research Applications
Biochemical Mechanisms and Insulin Secretion
One of the prominent applications of mono-methyl succinate is in the study of insulin secretion mechanisms. Research indicates that MMSucc stimulates insulin release from pancreatic beta-cells in a biphasic manner when combined with glucose. The stimulation is dependent on extracellular calcium influx, highlighting its potential as a pharmacological agent for diabetes management. Specifically, MMSucc was shown to enhance insulin secretion even at low glucose concentrations, suggesting its role in sensitizing beta-cells to glucose levels .
Key Findings:
- MMSucc activates phosphoinositide hydrolysis, contributing to insulin secretion.
- It enhances the effects of other compounds like forskolin, which increases cAMP levels in islets.
- The compound's ability to prime beta-cells for subsequent stimulation makes it a candidate for further diabetes research.
Pharmaceutical Applications
This compound is also recognized for its potential as a prodrug in pharmaceutical formulations. Notably, it has been investigated for its role in drug delivery systems targeting oxidative stress-related disorders. For instance, a recent study demonstrated that prodrugs releasing MMSucc can effectively treat chronic pain by targeting oxidative stress sites, thus minimizing side effects typically associated with conventional pain medications .
Case Study:
- Prodrug Mechanism: The α-ketoester 5b releases MMSucc upon exposure to peroxides, activating pathways that alleviate chronic pain.
- Clinical Relevance: This mechanism opens avenues for developing targeted therapies for conditions exacerbated by oxidative stress.
Organic Synthesis Intermediate
MMSucc serves as an important intermediate in organic synthesis and pharmaceutical synthesis. Its utility extends to the production of various chemical compounds, including colorants and fragrances. The compound is involved in reactions that yield other valuable chemical products, making it a versatile building block in synthetic chemistry .
Applications in Synthesis:
- Used as a substrate for synthesizing color candles that are smokeless and odorless.
- Acts as a precursor in the synthesis of more complex organic molecules.
Research and Development
The ongoing research into this compound encompasses various aspects of its biochemical properties and applications:
- Pharmacological Studies: Investigations into its effects on metabolic pathways and insulin sensitivity continue to reveal new therapeutic potentials.
- Chemical Synthesis Techniques: Advances in preparation methods have improved yield and purity, facilitating industrial-scale applications .
Data Table: Applications Overview
Application Area | Description | Key Findings/Notes |
---|---|---|
Biochemical Research | Insulin secretion modulation | Stimulates insulin release; enhances glucose sensitivity |
Pharmaceutical Formulations | Prodrug development for treating oxidative stress-related disorders | Targeted drug delivery; reduced side effects |
Organic Synthesis | Intermediate for synthesizing various compounds | Versatile building block; used in colorant production |
Research & Development | Ongoing studies on pharmacological effects and synthesis improvements | Continuous exploration of new therapeutic uses |
Mechanism of Action
The mechanism of action of 4-methoxy-4-oxobutanoate involves its interaction with specific molecular targets and pathways. For example, in the synthesis of HIV integrase inhibitors, the compound acts as a precursor that undergoes further chemical transformations to produce the active drug . The molecular targets include enzymes and receptors that are crucial for the biological activity of the final product .
Comparison with Similar Compounds
Structural Analogs
Succinic Acid Derivatives
- Key Insight : MMS and succinic anhydride share a biosynthetic relationship, but MMS exhibits unique bioactivity (e.g., metabolic stimulation) absent in the parent acid or anhydride .
Menthyl and Terpene Derivatives
- Rhodomollein XLIX: A grayanane diterpenoid containing a this compound group at C-2, distinguishing it from rhodojaponin VI. This substitution confers distinct pharmacological properties, such as antinociceptive effects .
Functional Analogs
Succinate Prodrugs
Compound | Structure | Function | Reference |
---|---|---|---|
MMS | CH3O-CO-CH2-CH2-COOH | Direct TCA cycle stimulation in β-cells | |
NV189 | Bis-(1-acetoxy-ethyl) succinate | Mitochondrial prodrug; bypasses Complex I deficiency |
- Key Insight : MMS acts as a natural metabolite, while NV189 is engineered for enhanced cell permeability. Both support mitochondrial energy production but differ in mechanism and application .
Acyl Glucosides/Glucuronides
- Mono-Methyl Substituted Acyl Glucoside: Exhibits similar degradation kinetics (t1/2 ≈16 h) to its glucuronide counterpart, suggesting comparable metabolic stability for MMS-derived esters .
Metabolic and Pharmacological Analogs
Metabolites in NADH Pathways
- Key Insight: MMS uniquely elevates NADH in ASD-Phen1 cells, unlike adenosine or succinamic acid, highlighting its role in subtype-specific metabolic dysregulation .
Succinate Dehydrogenase (SDH) Inhibitors
Compound | Target/Mechanism | Application | Reference |
---|---|---|---|
MMS | TCA cycle substrate | Metabolic rescue in β-cells | |
Pyrazole-4-carboxamide | SDH inhibition (fungicidal) | Agricultural antifungal agent |
- Key Insight : MMS supports SDH activity by providing succinate, whereas pyrazole derivatives inhibit SDH to block fungal growth .
Physicochemical and Stability Comparison
Property | MMS | Succinic Acid | NV189 |
---|---|---|---|
Water Solubility | Moderate | High | Low (prodrug) |
logP | ~0.5 (predicted) | -0.59 | 2.1 (estimated) |
Degradation t1/2 | N/A | Stable | >24 h |
- Key Insight : MMS balances moderate hydrophilicity and metabolic stability, making it suitable for acute cellular interventions .
Q & A
Basic Research Questions
Q. What are the standard synthetic routes for mono-methyl succinate, and what factors influence yield optimization?
this compound is commonly synthesized via esterification of succinic acid with methanol under acidic catalysis (e.g., sulfuric acid or p-toluenesulfonic acid). Key factors affecting yield include reactant molar ratios (typically 1:1.2 for succinic acid:methanol), temperature control (60–80°C), and catalyst concentration. Post-synthesis purification often involves recrystallization using solvents like ethyl acetate/hexane mixtures. Yield optimization requires monitoring reaction progress via thin-layer chromatography (TLC) and confirming purity through 1H-NMR (e.g., ester carbonyl signal at ~170 ppm) and GC-MS analysis .
Q. What spectroscopic techniques are recommended for characterizing this compound, and what key spectral markers should be observed?
Essential techniques include:
- 1H-NMR : Expect signals at δ 3.65 ppm (singlet, methyl ester group) and δ 2.60 ppm (multiplet, methylene protons adjacent to carbonyl groups).
- 13C-NMR : Peaks at ~170 ppm (ester carbonyl) and 52 ppm (methoxy carbon).
- IR Spectroscopy : Strong absorption at ~1740 cm−1 (C=O stretch). Cross-validate results with melting point analysis (56–59°C) and elemental composition data .
Q. What are the recommended storage conditions for this compound to ensure stability?
Store in airtight, light-resistant containers at 0–6°C to prevent hydrolysis. Desiccants like silica gel should be used to minimize moisture exposure. Periodic purity checks via HPLC (C18 column, acetonitrile/water mobile phase) are advised .
Advanced Research Questions
Q. How can researchers resolve discrepancies in reported kinetic parameters for enzyme-catalyzed reactions involving this compound?
Contradictions may arise from variations in enzyme sources (e.g., microbial vs. mammalian), substrate purity, or assay conditions (pH, temperature). Standardize protocols by:
- Using ultra-pure substrates (≥99% by HPLC).
- Validating enzyme activity via control reactions (e.g., succinate dehydrogenase assays).
- Applying Michaelis-Menten kinetics with triplicate measurements and statistical tools (ANOVA, R2 analysis) to assess reproducibility .
Q. What strategies mitigate matrix interference when quantifying this compound in biological samples via LC-MS?
To address ion suppression or co-elution:
- Sample Preparation : Solid-phase extraction (SPE) with mixed-mode sorbents (e.g., Oasis HLB) to isolate the analyte.
- Internal Standards : Deuterated this compound (e.g., d4-MMS) for isotope dilution.
- Chromatography : Use a HILIC column with 0.1% formic acid in acetonitrile/water to enhance separation. Validate method robustness via spike-recovery experiments (85–115% acceptable range) .
Q. How should experimental designs be structured to elucidate the metabolic pathways of this compound in microbial systems?
Combine isotopic tracing (13C-labeled MMS) with multi-omics approaches:
- Genomics : Knockout studies of succinate dehydrogenase (SDH) genes to identify rate-limiting steps.
- Metabolomics : LC-MS/MS profiling to track 13C-incorporation into TCA cycle intermediates.
- Flux Balance Analysis : Model carbon flux using software like COBRApy. Include negative controls (e.g., MMS-free media) and biological triplicates .
Q. Methodological Guidelines
- Data Contradiction Analysis : When conflicting results arise (e.g., variable enzymatic inhibition profiles), conduct sensitivity analyses to identify outlier datasets. Use Bland-Altman plots or principal component analysis (PCA) to assess methodological variability .
- Reproducibility : Document experimental parameters exhaustively (e.g., HPLC gradient profiles, NMR acquisition times) in supplementary materials to enable replication .
Properties
CAS No. |
214222-47-6 |
---|---|
Molecular Formula |
C5H7O4- |
Molecular Weight |
131.11 g/mol |
IUPAC Name |
4-methoxy-4-oxobutanoate |
InChI |
InChI=1S/C5H8O4/c1-9-5(8)3-2-4(6)7/h2-3H2,1H3,(H,6,7)/p-1 |
InChI Key |
JDRMYOQETPMYQX-UHFFFAOYSA-M |
Canonical SMILES |
COC(=O)CCC(=O)[O-] |
Origin of Product |
United States |
Disclaimer and Information on In-Vitro Research Products
Please be aware that all articles and product information presented on BenchChem are intended solely for informational purposes. The products available for purchase on BenchChem are specifically designed for in-vitro studies, which are conducted outside of living organisms. In-vitro studies, derived from the Latin term "in glass," involve experiments performed in controlled laboratory settings using cells or tissues. It is important to note that these products are not categorized as medicines or drugs, and they have not received approval from the FDA for the prevention, treatment, or cure of any medical condition, ailment, or disease. We must emphasize that any form of bodily introduction of these products into humans or animals is strictly prohibited by law. It is essential to adhere to these guidelines to ensure compliance with legal and ethical standards in research and experimentation.