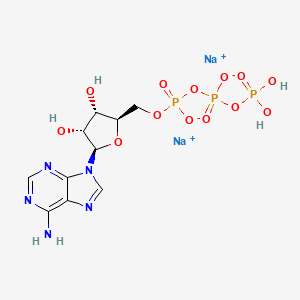
ATP (Adenosine-Triphosphate)
- Click on QUICK INQUIRY to receive a quote from our team of experts.
- With the quality product at a COMPETITIVE price, you can focus more on your research.
Overview
Description
Adenosine triphosphate disodium is a nucleotide that serves as a primary energy carrier in cells. It consists of three main components: adenine (a nitrogenous base), ribose (a sugar), and three phosphate groups. This compound is crucial for various cellular processes, including muscle contraction, nerve impulse propagation, and chemical synthesis .
Preparation Methods
Synthetic Routes and Reaction Conditions: Adenosine triphosphate disodium can be synthesized through enzymatic methods involving adenosine and polyphosphate. The process typically involves adenosine kinase and polyphosphate kinases in a one-pot reaction to achieve ATP regeneration and production .
Industrial Production Methods: On an industrial scale, adenosine triphosphate disodium is often produced using living yeast cells. This method is preferred due to its cost-effectiveness and scalability . The preparation involves dissolving disodium adenosine triphosphate in water for injection, adjusting the pH with arginine, and then sterilizing and packaging the solution .
Chemical Reactions Analysis
Types of Reactions: Adenosine triphosphate disodium undergoes various reactions, including hydrolysis, phosphorylation, and oxidation.
Common Reagents and Conditions:
Hydrolysis: This reaction involves the addition of water, breaking the bonds between phosphate groups and releasing energy.
Phosphorylation: This process transfers a phosphate group to another molecule, often mediated by enzymes.
Oxidation: In cellular respiration, the oxidation of NADH and FADH2 results in the synthesis of adenosine triphosphate.
Major Products: The primary products of these reactions include adenosine diphosphate (ADP) and adenosine monophosphate (AMP), which can be further regenerated into adenosine triphosphate .
Scientific Research Applications
Adenosine triphosphate disodium is widely used in scientific research due to its role as an energy carrier. Its applications include:
Chemistry: Used as a substrate in various enzymatic reactions and as a coenzyme in biochemical processes.
Biology: Essential for cellular processes such as respiration, biosynthetic reactions, and cell division.
Medicine: Investigated for its potential in treating conditions like heart disease, cancer, and hepatitis.
Industry: Utilized in the production of biopharmaceuticals and as a reagent in diagnostic assays.
Mechanism of Action
Adenosine triphosphate disodium stores and transports chemical energy within cells. It plays a crucial role in the synthesis of nucleic acids and is involved in various cellular processes, including muscle contraction and nerve impulse propagation . The compound is produced primarily in mitochondria through oxidative phosphorylation, catalyzed by ATP synthase .
Comparison with Similar Compounds
Adenosine Diphosphate (ADP): Contains two phosphate groups and is a product of adenosine triphosphate hydrolysis.
Adenosine Monophosphate (AMP): Contains one phosphate group and is a further product of adenosine triphosphate hydrolysis.
Guanosine Triphosphate (GTP): Similar in structure but contains guanine instead of adenine.
Uniqueness: Adenosine triphosphate disodium is unique due to its role as the primary energy carrier in cells. Its ability to store and release energy efficiently makes it indispensable for various cellular functions .
Properties
Molecular Formula |
C10H14N5Na2O13P3 |
---|---|
Molecular Weight |
551.14 g/mol |
IUPAC Name |
disodium;[[(2R,3S,4R,5R)-5-(6-aminopurin-9-yl)-3,4-dihydroxyoxolan-2-yl]methoxy-oxidophosphoryl] phosphono phosphate |
InChI |
InChI=1S/C10H16N5O13P3.2Na/c11-8-5-9(13-2-12-8)15(3-14-5)10-7(17)6(16)4(26-10)1-25-30(21,22)28-31(23,24)27-29(18,19)20;;/h2-4,6-7,10,16-17H,1H2,(H,21,22)(H,23,24)(H2,11,12,13)(H2,18,19,20);;/q;2*+1/p-2/t4-,6-,7-,10-;;/m1../s1 |
InChI Key |
TTWYZDPBDWHJOR-IDIVVRGQSA-L |
Isomeric SMILES |
C1=NC(=C2C(=N1)N(C=N2)[C@H]3[C@@H]([C@@H]([C@H](O3)COP(=O)([O-])OP(=O)([O-])OP(=O)(O)O)O)O)N.[Na+].[Na+] |
Canonical SMILES |
C1=NC(=C2C(=N1)N(C=N2)C3C(C(C(O3)COP(=O)([O-])OP(=O)([O-])OP(=O)(O)O)O)O)N.[Na+].[Na+] |
Origin of Product |
United States |
Disclaimer and Information on In-Vitro Research Products
Please be aware that all articles and product information presented on BenchChem are intended solely for informational purposes. The products available for purchase on BenchChem are specifically designed for in-vitro studies, which are conducted outside of living organisms. In-vitro studies, derived from the Latin term "in glass," involve experiments performed in controlled laboratory settings using cells or tissues. It is important to note that these products are not categorized as medicines or drugs, and they have not received approval from the FDA for the prevention, treatment, or cure of any medical condition, ailment, or disease. We must emphasize that any form of bodily introduction of these products into humans or animals is strictly prohibited by law. It is essential to adhere to these guidelines to ensure compliance with legal and ethical standards in research and experimentation.