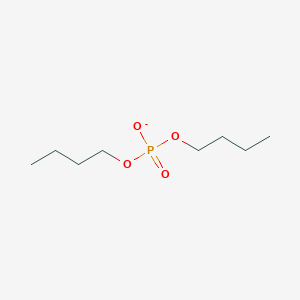
dibutyl phosphate
- Click on QUICK INQUIRY to receive a quote from our team of experts.
- With the quality product at a COMPETITIVE price, you can focus more on your research.
Overview
Description
Dibutyl phosphate (DBP), CAS 107-66-4, is an organophosphate compound characterized by its light amber color, odorless nature, and moderate acidity (pKa ~1.5–2.0). Key physical properties include a melting point of -13°C, boiling point of 135–138°C, density of 1.06 g/cm³, and water solubility of 18 g/L at 20°C . DBP is stable in neutral, acidic, and alkaline solutions but reacts with metals to produce flammable hydrogen gas. It is classified as inherently biodegradable, with an estimated atmospheric half-life of 4.99 years .
Preparation Methods
Synthetic Routes and Reaction Conditions
dibutyl phosphate can be synthesized through several methods. One common synthetic route involves the reaction of phosphorus oxychloride with butanol in the presence of a base such as triethylamine. The reaction typically proceeds under reflux conditions, and the product is purified through distillation or recrystallization.
Another method involves the transesterification of dibutyl phosphite with butanol. This reaction is catalyzed by acids or bases and is carried out under mild conditions to yield dibutoxyphosphinate.
Industrial Production Methods
In industrial settings, dibutoxyphosphinate is produced on a larger scale using continuous flow reactors. These reactors allow for precise control of reaction conditions, leading to higher yields and purity of the final product. The use of catalysts and optimized reaction parameters further enhances the efficiency of the production process.
Chemical Reactions Analysis
Acid-Base Reactions
DBP acts as a moderately strong acid (pKa ≈ 1.53 in water) due to its P–OH group, enabling exothermic neutralization with bases . For example:
C8H19O4P+NaOH→C8H18O4PNa+H2O+Heat
Reactions with strong bases (e.g., alkali metals) produce flammable hydrogen gas :
2DBP+2Na→2DBP-Na+H2↑
Thermal Decomposition
At elevated temperatures (>135°C), DBP decomposes exothermically, releasing toxic fumes of phosphoric acid and phosphorus oxides :
C8H19O4PΔH3PO4+C4H9OH+C4H8+COx
Key decomposition products :
-
Phosphoric acid (H3PO4)
-
1-Butene (C4H8)
-
Carbon oxides (CO,CO2)
Acid-Catalyzed Hydrolysis
Under acidic conditions, DBP undergoes ester cleavage to form mono-butyl phosphate and butanol :
C8H19O4P+H2OH+C4H9H2O4P+C4H9OH
Enzymatic Hydrolysis
Sphingobium PTE enzyme catalyzes hydrolysis of DBP derivatives (e.g., dibutyl phenyl phosphate) with a solvent isotope effect (kH2O/kD2O) of ~2.0, indicating proton transfer in the rate-limiting step .
Substrate | Enzyme | kcat (s−1) | pH Optimum |
---|---|---|---|
Dibutyl phenyl phosphate | Sb-PTE | 0.15 ± 0.02 | 10.0 |
Solvent Effects on Reactivity
31P NMR studies reveal solvent-dependent dissociation of DBP :
Solvent | Dielectric Constant (ε) | pKa |
---|---|---|
Water | 80.1 | 1.53 |
Methanol | 32.7 | 2.8 |
Ethanol | 24.3 | 3.2 |
Higher ε solvents enhance ionization of the P–OH group. Ion association with tetramethylammonium (TMA+) occurs with Kc=0.49M−1, indicating weak interactions .
Degradation Pathways
DBP forms as a degradation product of tributyl phosphate (TBP) in nitric acid solutions. Rates increase with temperature and acid concentration :
Temperature (°C) | [HNO3] (M) | DBP Formation Rate (%/day) |
---|---|---|
25 | 1.0 | 0.0003 |
70 | 5.0 | 0.090 |
Reactivity with Metals
DBP reacts with metals (e.g., iron, aluminum) to form metal phosphates and hydrogen gas :
2DBP+2Fe→2Fe(DBP)+H2↑
Oxidation Reactions
Strong oxidizing agents (e.g., KMnO4) oxidize DBP, producing phosphoric acid and butyric acid derivatives :
C8H19O4P+[O]→H3PO4+C3H7COOH+H2O
Scientific Research Applications
2.1. Catalyst in Paints and Coatings
Dibutyl phosphate serves as a catalyst for cross-linking in the paint industry, enhancing the durability and performance of coatings. Its application in this sector accounts for a significant portion of its usage, particularly in Japan and Germany, where production volumes have been reported at 150-250 tonnes annually during the early 1990s .
2.2. Plasticizers and Hydraulic Fluids
In addition to its role in paints, this compound is used as a plasticizer in various polymers, improving flexibility and processability. It also finds applications as a hydraulic fluid due to its stability and low volatility .
2.3. Antifoaming Agent
DBP is employed as an antifoaming agent in industrial processes such as ore separation, where it helps reduce foam formation that can interfere with operations .
2.4. Heat Exchange Dielectric Medium
The compound is utilized in heat exchange systems as a dielectric medium, which is crucial for efficient thermal management in electrical applications .
3.1. Toxicological Studies
Research has highlighted potential health effects associated with this compound exposure. Studies indicate that it can cause skin and eye irritation in animal models . Long-term exposure assessments have shown low potential risks; however, certain studies noted delayed neurotoxicity after prolonged dermal applications in chickens .
3.2. Role in Drug Delivery Systems
This compound has been investigated for its role in drug dispersion within medicinal patches, enhancing the delivery of peptide hormones through controlled-release mechanisms . This application underscores its significance in pharmaceutical formulations.
Case Studies
Mechanism of Action
The mechanism by which dibutoxyphosphinate exerts its effects involves its interaction with molecular targets such as enzymes and receptors. The butoxy groups enhance its lipophilicity, allowing it to penetrate biological membranes and interact with intracellular targets. The phosphorus atom can form covalent bonds with nucleophilic sites on proteins, leading to inhibition or modification of their activity.
Comparison with Similar Compounds
Comparative Analysis with Similar Compounds
Tributyl Phosphate (TBP)
Key Differences :
- TBP’s lower water solubility and higher hydrophobicity make it more effective in solvent extraction processes, particularly in nuclear industries. However, its environmental persistence raises ecological concerns compared to DBP .
Dibutyl Phenyl Phosphate (DBPP)
Key Differences :
- DBPP’s aromatic structure enhances thermal stability, making it suitable for high-temperature applications. Its faster biodegradation in aquatic environments (3.5 days) contrasts with DBP’s slower atmospheric degradation .
Monofluorophosphate
Key Differences :
- Monofluorophosphate’s fluoride group increases susceptibility to hydrolysis, limiting its utility in aqueous systems. DBP’s stability makes it preferable as a chromatographic reference .
Butyl Dibutylphosphinate
Property | This compound (DBP) | Butyl Dibutylphosphinate |
---|---|---|
Solubility Minimum | Not reported | 73°C |
Hydrogen Bonding | Moderate donor | Non-donor |
Applications | Catalysis, extraction | Specialty solvent |
Key Differences :
- The absence of hydrogen-bonding capacity in butyl dibutylphosphinate reduces its interaction with polar solvents, favoring non-aqueous applications compared to DBP .
Q & A
Basic Research Questions
Q. What are the primary methods for synthesizing and characterizing dibutyl phosphate (DBP) in laboratory settings?
DBP is synthesized via esterification of phosphoric acid with n-butanol under acidic catalysis. Key characterization methods include:
- Nuclear Magnetic Resonance (NMR) : To confirm esterification by analyzing shifts in 31P-NMR (e.g., δ = -0.5 to -1.5 ppm for DBP) .
- Fourier-Transform Infrared Spectroscopy (FTIR) : Identification of P=O stretching (~1250 cm−1) and P-O-C vibrations (~1050 cm−1) .
- Titration : Quantification of acidic protons (pKa ~1.5–2.5) using NaOH .
Q. How does DBP solubility in aqueous solutions influence its applications in solvent extraction systems?
DBP exhibits limited water solubility (18 g/L at 20°C) but forms micelles or ion pairs in acidic/basic media, enhancing its utility in liquid-liquid extraction. For example:
- In HNO3 solutions, DBP coordinates with uranyl ions (UO22+) to form uranyl this compound complexes, critical for nuclear fuel reprocessing .
- Solubility decreases with increasing nitrate concentration due to salting-out effects .
Q. What safety protocols are essential for handling DBP in laboratory environments?
- Personal Protective Equipment (PPE) : Acid-resistant gloves, goggles, and lab coats (DBP is corrosive and irritates eyes/mucous membranes) .
- Ventilation : Use fume hoods to avoid inhalation of decomposition products (e.g., POx fumes at >188°C) .
- Waste Disposal : Neutralize with alkaline solutions (e.g., 10% NaOH) before disposal to mitigate environmental persistence (half-life: ~4.99 years in air) .
Q. What role does DBP play in polymer science, particularly as a catalyst or additive?
DBP acts as:
- Crosslinking Catalyst : Accelerates curing in polyurethane and epoxy resins by donating acidic protons to initiate polymerization .
- Antistatic Agent : Reduces static charge accumulation in textiles via surface adsorption of phosphate groups .
Advanced Research Questions
Q. How do degradation products of DBP (e.g., monobutyl phosphate) affect solvent extraction efficiency in nuclear waste processing?
- HDBP (this compound) : Forms stable complexes with Zr4+ and UO22+, increasing organic-phase retention and reducing separation efficiency .
- Monobutyl Phosphate (MBP) : Enhances interfacial crud formation in TBP-based systems, requiring frequent solvent cleanup .
- Mitigation Strategy : Use of scrubbing agents (e.g., Na2CO3) to strip degradation products from organic phases .
Q. What experimental approaches quantify DBP’s impact on microbial growth in environmental toxicity studies?
- Clostridium acetobutylicum Assay : DBP at 0.1–1.0 mM increases dry cell mass (0.63 → 0.94 g/L) by altering membrane permeability to nutrients .
- Respirometry : Measure O2 consumption rates to assess inhibitory effects on aerobic microbes .
Q. How does DBP interact with transition metals (e.g., Fe3+, Zr4+) in aqueous/organic biphasic systems?
- Ferric-DBP Complexes : Insoluble in 30% TBP-Amsco solutions, precipitating as Fe(DBP)3 (solubility <0.01 g/L in 3M HNO3) .
- Zirconium-DBP Coordination : Forms ZrO(DBP)2 precipitates, complicating nuclear fuel cycle operations .
Q. What analytical challenges arise when detecting DBP in complex matrices (e.g., nitrate-rich nuclear waste)?
- Matrix Interference : High nitrate/nitrite concentrations mask DBP signals in traditional chromatographic methods .
- Advanced Techniques :
- Ion-Pair Chromatography : Tetrahexylammonium bromide improves DBP resolution (retention time: 8–10 min) .
- Isotachophoresis : Separates DBP from nitrate via electrophoretic mobility differences (detection limit: 0.1 ppm) .
Q. How does DBP influence the thermodynamics of actinide separation in nuclear reprocessing?
- Uranyl Extraction : DBP enhances UO22+ distribution ratios (DU) in low-acidity media but reduces selectivity over Pu4+ due to competing complexation .
- Temperature Dependence : Solubility of uranyl-DBP complexes decreases by 30% at 50°C compared to 24°C .
Q. What methodologies address DBP’s environmental persistence in biodegradation studies?
Properties
Molecular Formula |
C8H18O4P- |
---|---|
Molecular Weight |
209.20 g/mol |
IUPAC Name |
dibutyl phosphate |
InChI |
InChI=1S/C8H19O4P/c1-3-5-7-11-13(9,10)12-8-6-4-2/h3-8H2,1-2H3,(H,9,10)/p-1 |
InChI Key |
JYFHYPJRHGVZDY-UHFFFAOYSA-M |
Canonical SMILES |
CCCCOP(=O)([O-])OCCCC |
physical_description |
Liquid |
Origin of Product |
United States |
Disclaimer and Information on In-Vitro Research Products
Please be aware that all articles and product information presented on BenchChem are intended solely for informational purposes. The products available for purchase on BenchChem are specifically designed for in-vitro studies, which are conducted outside of living organisms. In-vitro studies, derived from the Latin term "in glass," involve experiments performed in controlled laboratory settings using cells or tissues. It is important to note that these products are not categorized as medicines or drugs, and they have not received approval from the FDA for the prevention, treatment, or cure of any medical condition, ailment, or disease. We must emphasize that any form of bodily introduction of these products into humans or animals is strictly prohibited by law. It is essential to adhere to these guidelines to ensure compliance with legal and ethical standards in research and experimentation.