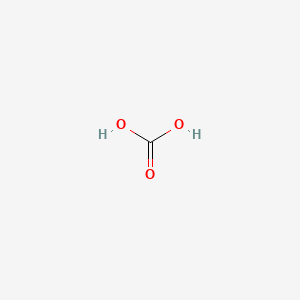
Carbonic Acid
Overview
Description
Carbonic acid (H₂CO₃) is a weak, diprotic acid formed by the dissolution of carbon dioxide (CO₂) in water. It plays critical roles in biological systems (e.g., the bicarbonate buffer system in blood), geochemical processes (e.g., ocean acidification), and industrial applications (e.g., carbonation of beverages) . Despite its ubiquity, pure this compound is notoriously unstable under standard conditions, decomposing into CO₂ and H₂O. Its isolation requires extreme conditions, such as high pressure (e.g., in icy satellites) or cryogenic matrix isolation .
Scientific Research Applications
Food and Beverage Industry
Carbonated Beverages
- Carbonic acid is essential in the production of carbonated drinks, such as sodas and sparkling waters. The carbonation process involves dissolving carbon dioxide in water, which forms this compound, giving beverages their characteristic fizz.
Beverage Type | This compound Role |
---|---|
Soft Drinks | Provides carbonation and flavor |
Sparkling Wines | Enhances effervescence |
Mineral Waters | Naturally occurring this compound |
Food Processing
- In food processing, this compound is used for its preservative qualities and to enhance the texture of frozen products. It helps maintain the quality of refrigerated goods by inhibiting microbial growth.
Medical Applications
Therapeutic Uses
- This compound is utilized in therapeutic baths, particularly for patients with hypertension or cardiovascular issues. The gas is absorbed through the skin, promoting relaxation and improving circulation.
Vomiting Induction
- In emergency medicine, this compound solutions are sometimes ingested to induce vomiting in cases of drug overdose, though this practice requires careful medical supervision due to potential risks.
Environmental Applications
Carbon Capture and Storage
- This compound plays a crucial role in carbon capture technologies aimed at reducing atmospheric CO2 levels. By converting CO2 into a more stable form, it facilitates the storage of carbon dioxide underground, mitigating climate change impacts.
Ocean Acidification Studies
- Research on this compound contributes to understanding ocean chemistry and its role in buffering ocean pH levels against acidification caused by increased atmospheric CO2 concentrations. This knowledge is vital for marine ecosystem health.
Industrial Applications
Water Treatment
- This compound is used in water treatment processes to adjust pH levels and enhance the solubility of minerals, making it easier to remove impurities from water supplies.
Enhanced Oil Recovery
- In the oil industry, carbon dioxide (which forms this compound when dissolved in water) is injected into oil fields to increase hydrocarbon recovery rates. This method has been employed since the 1950s and has significantly improved oil extraction efficiency.
Industry | Application |
---|---|
Oil Industry | Enhanced oil recovery |
Water Treatment | pH adjustment and mineral solubility |
Food Processing | Preservation and texture enhancement |
Cosmetic Applications
Skin Treatments
- In cosmetology, this compound is incorporated into skin treatments for its exfoliating properties. It aids in cleansing the skin and promoting rejuvenation through controlled chemical reactions.
Case Study 1: Carbon Capture Technology
A recent study highlighted advancements in capturing atmospheric CO2 through chemical processes involving this compound. The research demonstrated that utilizing this compound can enhance mineralization processes, effectively storing CO2 as stable mineral compounds underground .
Case Study 2: Medical Use of Carbon Dioxide Baths
Health resorts have reported positive outcomes from using carbon dioxide baths enriched with this compound for patients with cardiovascular conditions. Patients experienced improved blood circulation and reduced symptoms of hypertension after regular treatments .
Case Study 3: Enhanced Oil Recovery Techniques
The Weyburn oilfield project in Canada exemplifies successful implementation of CO2 injection for enhanced oil recovery. Since its inception, this project has led to significant increases in oil production while simultaneously storing millions of tons of CO2 underground .
Chemical Reactions Analysis
Formation of Carbonic Acid
This compound forms via the reversible reaction of CO₂ with water:
This reaction is critical in ocean acidification, where dissolved CO₂ increases H₂CO₃ levels, lowering seawater pH .
Key Observations :
-
The reaction is exothermic but proceeds with high activation barriers in the gas phase (51.40 kcal/mol for CO₂–H₂O, decreasing to 19.84 kcal/mol with three water molecules) .
-
Metal centers (e.g., Al(OH)₃) significantly lower activation barriers and alter reaction mechanisms, enabling efficient H₂CO₃ formation .
-
In aqueous environments, CO₂ hydration involves rapid proton transfer steps, stabilized by hydrogen bonding and solvent interactions .
Dissociation Equilibria
This compound rapidly dissociates into bicarbonate (HCO₃⁻) and protons:
A second dissociation step produces carbonate (CO₃²⁻):
Table 1: Dissociation Constants of this compound
Reaction | p*Kₐ Value | Source |
---|---|---|
H₂CO₃ ⇌ H⁺ + HCO₃⁻ | 3.49 ± 0.05 | |
HCO₃⁻ ⇌ H⁺ + CO₃²⁻ | ~10.3 |
Protonation Reactions
This compound acts as an effective protonating agent, even in biological systems:
-
Kinetic Dominance : Protonation by H₂CO₃ occurs faster than decomposition into CO₂ and H₂O, making it a key player in physiological proton fluxes .
-
Free Energy Correlation : Proton transfer rates correlate with Δp*Kₐ values between H₂CO₃ and conjugate bases (e.g., carboxylates) .
-
Biological Significance : In blood plasma, H₂CO₃ contributes ~70× more to protonation than aqueous H⁺ ions, challenging traditional views of acid-base regulation .
Environmental and Biological Roles
-
Ocean Acidification : Rising atmospheric CO₂ increases H₂CO₃, reducing seawater pH and carbonate saturation, impacting marine ecosystems .
-
Physiological pH Regulation : H₂CO₃ mediates proton transfer in biological fluids, with implications for acidosis and metabolic processes .
-
Geochemical Cycling : this compound facilitates CO₂ mineralization in natural waters and industrial CO₂ capture systems .
Structural and Mechanistic Insights
-
Hydration Structure : CO₂ solvation involves weak hydrogen bonding with water molecules, creating a transient hydrophobic environment .
-
Metal-Catalyzed Formation : Al(OH)₃ coordination lowers activation barriers by stabilizing transition states, enabling efficient H₂CO₃ production .
-
Spectroscopic Evidence : IR and fluorescence studies confirm rapid proton transfer kinetics, validating H₂CO₃’s role as a reactive acid .
Challenges and Controversies
-
Thermodynamic vs. Kinetic Stability : While H₂CO₃ is thermodynamically unstable (rapidly decomposes), its transient existence allows significant chemical reactivity .
-
p*Kₐ Discrepancy : The apparent pKₐ (6.35) vs. the intrinsic pKₐ (3.49) highlights the need to distinguish equilibrium constants involving CO₂ gas from pure aqueous dissociation .
Q & A
Basic Research Questions
Q. How are the acid dissociation constants (pKa₁ and pKa₂) of carbonic acid experimentally determined in aqueous systems?
Methodological Answer: The pKa values are typically derived using pH titration combined with the Bjerrum plot method, which correlates pH-dependent speciation of this compound, bicarbonate (HCO₃⁻), and carbonate (CO₃²⁻). Spectroscopic techniques like NMR or infrared (IR) spectroscopy validate equilibrium concentrations under controlled ionic strength and temperature .
Q. What experimental approaches are used to study the bicarbonate buffer system in physiological contexts?
Methodological Answer: Researchers employ stopped-flow kinetics to measure CO₂ hydration rates catalyzed by carbonic anhydrase in red blood cells. Simultaneous pH and ion-selective electrode measurements monitor real-time changes in HCO₃⁻/CO₂ ratios, while computational models integrate these data to predict buffering capacity in blood plasma .
Q. How can this compound’s role in ocean acidification be quantified in laboratory settings?
Methodological Answer: Use closed-system reactors to simulate seawater conditions, varying pCO₂ levels via gas mixing. Total inorganic carbon (TIC) and alkalinity are measured via potentiometric titrations, while Raman spectroscopy tracks carbonate ion (CO₃²⁻) depletion. Data are cross-validated with Henry’s law constants for CO₂ solubility .
Advanced Research Questions
Q. What spectroscopic techniques resolve this compound’s hydration dynamics and short-lived intermediates?
Methodological Answer: X-ray absorption spectroscopy (XAS) at synchrotron facilities (e.g., Advanced Light Source) captures hydration shells around aqueous this compound. Complementary ab initio molecular dynamics simulations model hydrogen-bonding networks, resolving transient species like H₃O⁺/HCO₃⁻ ion pairs .
Q. How do researchers address contradictions in reported stability of this compound across phases (gas, aqueous, solid)?
Methodological Answer: Matrix isolation techniques at cryogenic temperatures (e.g., argon matrices at 10 K) stabilize this compound for IR characterization. Gas-phase studies use supersonic jet expansions coupled with cavity ring-down spectroscopy to detect elusive conformers, reconciling discrepancies with theoretical predictions .
Q. What computational strategies improve kinetic models of CO₂ hydration/dehydration in non-equilibrium systems?
Methodological Answer: Density functional theory (DFT) calculates activation barriers for CO₂ + H₂O → H₂CO₃, while machine learning algorithms train on experimental rate constants (e.g., from stopped-flow UV-Vis) to predict pH-dependent kinetics in heterogeneous environments like biofilms .
Q. How are α- and β-crystalline forms of this compound differentiated and characterized?
Methodological Answer: Low-temperature X-ray diffraction identifies polymorphic structures, with α-carbonic acid forming monoclinic crystals and β-carbonic acid adopting orthorhombic symmetry. Polarized Raman spectroscopy distinguishes H-bonding patterns, validated against computed vibrational spectra .
Q. What methodologies isolate gas-phase this compound for extraterrestrial chemistry studies?
Methodological Answer: Supersonic nozzle expansions coupled with laser-induced fluorescence or high-resolution IR spectroscopy detect gas-phase H₂CO₃. These setups mimic low-pressure environments (e.g., Martian poles), with spectral data aiding identification in astronomical observations .
Q. How do researchers reconcile conflicting data on this compound’s catalytic role in prebiotic amino acid synthesis?
Methodological Answer: Isotopic labeling (e.g., ¹³C/¹⁸O) tracks carboxylation pathways in simulated hydrothermal vents. Liquid chromatography-mass spectrometry (LC-MS) identifies reaction intermediates, while quantum mechanics/molecular mechanics (QM/MM) simulations validate pH-dependent reaction coordinates .
Q. What experimental models elucidate carbonic anhydrase II’s role in autoimmune pancreatitis?
Methodological Answer: Neonatally thymectomized mice immunized with carbonic anhydrase II (CA-II) develop pancreatic inflammation. Flow cytometry quantifies CD4⁺ T-cell infiltration, while TUNEL assays and cytokine profiling (IFN-γ/IL-4) differentiate Th1-driven pathology from other mechanisms .
Comparison with Similar Compounds
Key Properties :
- Molecular Formula : H₂CO₃
- Molar Mass : 62.03 g/mol
- Acidity : pKa₁ = 6.36, pKa₂ = 10.3 (in aqueous solution)
- Stability : Metastable under high pressure (>1 GPa) or at temperatures below −30°C .
Carbonic acid shares structural and functional similarities with several compounds, including esters, other acids, and conjugate bases. Below is a detailed analysis:
This compound Esters: Monoethyl Ester (CAEE) and Monomethyl Ester (CAME)
This compound esters, such as this compound monoethyl ester (CAEE) and monomethyl ester (CAME), are derivatives where one hydroxyl group of H₂CO₃ is replaced by an alkoxy group. These compounds exhibit greater stability than this compound and can be isolated as solids under standard conditions .
Key Differences :
Research Findings :
- FTIR spectra of CAME and CAEE lack the characteristic O–H stretches of β-H₂CO₃, confirming their esterified structures .
- Controversy exists over the α-polymorph of H₂CO₃, which some studies suggest is actually CAME .
Formic Acid (HCOOH) and Sulfurous Acid (H₂SO₃)
Both formic acid (a monocarboxylic acid) and sulfurous acid (a diprotic acid) share functional groups with H₂CO₃ but differ in stability and reactivity.
Comparison :
Research Findings :
- Hydration clusters of H₂CO₃ exhibit stronger hydrogen-bonding networks than HCOOH, enhancing its stability in aqueous environments .
Carbamic Acid (H₂NCOOH) and Derivatives
Carbamic acid, a carbamate derivative, shares a carbonyl group with H₂CO₃ but includes an amine group. Its esters are studied for pharmacological applications.
Pharmacological Activity :
- Carbamic and this compound esters (e.g., PS-1, P-8-Y) show central nervous system (CNS) excitation and antidepressant effects, with lower toxicity than imipramine .
Bicarbonate (HCO₃⁻) and Carbonate (CO₃²⁻)
These conjugate bases of H₂CO₃ are critical in buffering systems:
- Bicarbonate Buffer System : Maintains blood pH via equilibrium: CO₂ + H₂O ⇌ H₂CO₃ ⇌ H⁺ + HCO₃⁻ .
- Ocean Acidification : Increased CO₂ dissolution lowers ocean pH, shifting equilibrium toward H₂CO₃ and reducing carbonate ion availability .
Astrophysics and Planetary Science
- This compound forms in icy satellites (e.g., Europa) under high pressure, insulating water ice from rocky cores and altering chemical models of celestial bodies .
- Gas-phase H₂CO₃ exists in comets and Mars’ atmosphere, detected via sublimation studies .
Catalysis and Materials Science
- Nitrogen-doped carbonaceous materials derived from H₂CO₃ show promise in redox catalysis and acid/base reactions .
Medical Therapeutics
- Carbonic anhydrase inhibitors (e.g., thiourea derivatives) target isoforms hCA I/II, with inhibition constants (Kᵢ) as low as 3.4 μM .
Preparation Methods
Acid-Carbonate Reaction
The most traditional method for generating carbonic acid involves the reaction of strong acids with calcium carbonate (CaCO₃). When hydrochloric acid (HCl) or sulfuric acid (H₂SO₄) reacts with calcium carbonate, carbon dioxide (CO₂) is liberated and subsequently dissolved in water to form this compound :
3 + 2\text{HCl} \rightarrow \text{CaCl}2 + \text{CO}2 + \text{H}2\text{O} \quad \text{and} \quad \text{CO}2 + \text{H}2\text{O} \rightleftharpoons \text{H}2\text{CO}3
This method is widely used in educational laboratories due to its simplicity . However, the equilibrium favors CO₂ and water, necessitating low temperatures or pressurized systems to enhance H₂CO₃ yield. For instance, cooling the reaction mixture to 0–5°C increases solubility, but the acid remains prone to decomposition .
Gas Dissolution and Aqueous Formation
Direct dissolution of CO₂ in water under controlled conditions forms this compound, albeit transiently. The equilibrium constant for this reaction () is approximately at 25°C, reflecting the low stability of H₂CO₃ in aqueous solutions . Recent high-pressure studies (2.4–3.0 GPa) at 110°C demonstrated that neutral H₂CO₃ becomes a significant species in CO₂-saturated water, persisting for hours due to slowed decomposition kinetics . Raman spectroscopy confirmed distinct vibrational modes at 1,040 cm⁻¹ (C–O symmetric stretch) and 1,450 cm⁻¹ (O–H bending) under these conditions .
Table 1: Conditions for Stabilizing Aqueous this compound
Pressure (GPa) | Temperature (°C) | H₂CO₃ Concentration (mol/L) | Stability Duration |
---|---|---|---|
2.4 | 110 | 0.15–0.20 | 2–4 hours |
3.0 | 110 | 0.25–0.30 | 6–8 hours |
Protonation of Bicarbonate Salts
This compound can be synthesized via protonation of bicarbonate (HCO₃⁻) in acidic media. A cryogenic technique involving rapid freezing of bicarbonate solutions acidified with HCl at -30°C yielded thin films of H₂CO₃, characterized by FTIR spectroscopy . Key absorption bands at 1,690 cm⁻¹ (C=O stretch) and 2,520 cm⁻¹ (O–H stretch) confirmed its formation . Buffered systems at pH 7.6 ± 0.2 minimize HCO₃⁻ decomposition, enabling transient detection of H₂CO₃ at physiological conditions .
Novel Catalytic Cyclopropanation Route
A 2022 patent disclosed a groundbreaking method using diazoacetic acid ethyl ester (C₃H₅N₂O₂) and isopentenyl acid ester (C₅H₈O₂) with a salen cobalt catalyst . This two-step process avoids hazardous oxidants:
-
Cyclopropanation : The cobalt catalyst (0.5–3 mol%) facilitates double-bond addition at 10–90°C in dichloromethane or toluene, forming a cyclopropyl intermediate .
-
Hydrolysis : The intermediate undergoes alkaline hydrolysis in methanol or ethanol at 10–60°C to yield caronic acid (C₆H₁₀O₄), a stable analog of H₂CO₃ .
Table 2: Optimal Conditions for Cyclopropanation
Parameter | Range |
---|---|
Catalyst loading | 0.005–0.03 mol eq. |
Reaction temperature | 10–90°C |
Solvent | Dichloromethane |
Hydrolysis time | 0.5–12 hours |
This method achieves 85–92% yield with negligible environmental waste, making it suitable for industrial scale-up .
High-Pressure and High-Temperature Synthesis
Laser-assisted heating of CO₂/H₂O mixtures at 3.0 GPa and 300°C produced solid H₂CO₃, as verified by X-ray diffraction . The crystalline phase exhibited a monoclinic structure (space group P2₁/c), with lattice parameters a = 5.42 Å, b = 6.78 Å, and c = 7.15 Å . This method mimics conditions in subduction zones, suggesting H₂CO₃ may play a role in deep Earth carbon cycling .
Matrix Isolation and Cryogenic Techniques
Matrix isolation in argon at 10 K enabled the spectroscopic characterization of this compound vapor sublimed from β-H₂CO₃ crystals . FTIR analysis revealed monomeric H₂CO₃ with vibrational frequencies at 1,120 cm⁻¹ (C–O) and 1,980 cm⁻¹ (O–H), distinct from its polymeric forms . This technique is pivotal for studying H₂CO₃’s gas-phase properties without interference from decomposition products.
Properties
CAS No. |
22719-67-1 |
---|---|
Molecular Formula |
H2CO3 CH2O3 |
Molecular Weight |
62.025 g/mol |
IUPAC Name |
carbonic acid |
InChI |
InChI=1S/CH2O3/c2-1(3)4/h(H2,2,3,4) |
InChI Key |
BVKZGUZCCUSVTD-UHFFFAOYSA-N |
SMILES |
C(=O)(O)O |
Canonical SMILES |
C(=O)(O)O |
melting_point |
720 °C |
physical_description |
Solid |
solubility |
12.8 mg/mL (cold water) |
Origin of Product |
United States |
Synthesis routes and methods I
Procedure details
Synthesis routes and methods II
Procedure details
Synthesis routes and methods III
Procedure details
Synthesis routes and methods IV
Procedure details
Synthesis routes and methods V
Procedure details
Retrosynthesis Analysis
AI-Powered Synthesis Planning: Our tool employs the Template_relevance Pistachio, Template_relevance Bkms_metabolic, Template_relevance Pistachio_ringbreaker, Template_relevance Reaxys, Template_relevance Reaxys_biocatalysis model, leveraging a vast database of chemical reactions to predict feasible synthetic routes.
One-Step Synthesis Focus: Specifically designed for one-step synthesis, it provides concise and direct routes for your target compounds, streamlining the synthesis process.
Accurate Predictions: Utilizing the extensive PISTACHIO, BKMS_METABOLIC, PISTACHIO_RINGBREAKER, REAXYS, REAXYS_BIOCATALYSIS database, our tool offers high-accuracy predictions, reflecting the latest in chemical research and data.
Strategy Settings
Precursor scoring | Relevance Heuristic |
---|---|
Min. plausibility | 0.01 |
Model | Template_relevance |
Template Set | Pistachio/Bkms_metabolic/Pistachio_ringbreaker/Reaxys/Reaxys_biocatalysis |
Top-N result to add to graph | 6 |
Feasible Synthetic Routes
Disclaimer and Information on In-Vitro Research Products
Please be aware that all articles and product information presented on BenchChem are intended solely for informational purposes. The products available for purchase on BenchChem are specifically designed for in-vitro studies, which are conducted outside of living organisms. In-vitro studies, derived from the Latin term "in glass," involve experiments performed in controlled laboratory settings using cells or tissues. It is important to note that these products are not categorized as medicines or drugs, and they have not received approval from the FDA for the prevention, treatment, or cure of any medical condition, ailment, or disease. We must emphasize that any form of bodily introduction of these products into humans or animals is strictly prohibited by law. It is essential to adhere to these guidelines to ensure compliance with legal and ethical standards in research and experimentation.