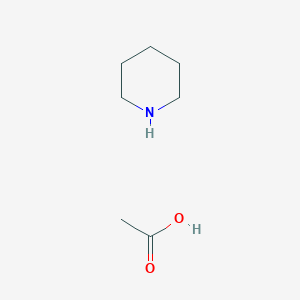
Piperidinium acetate
Overview
Description
Piperidinium acetate is an organic compound that consists of a piperidine ring bonded to an acetate group. Piperidine is a six-membered heterocyclic amine with the molecular formula C5H11N, while acetate is the conjugate base of acetic acid with the formula CH3COO-. This compound is commonly used in organic synthesis and as a building block for various pharmaceuticals and agrochemicals.
Preparation Methods
Synthetic Routes and Reaction Conditions
Piperidinium acetate can be synthesized through several methods. One common method involves the reaction of piperidine with acetic anhydride or acetyl chloride. The reaction typically occurs under mild conditions and yields piperidine, acetate as the primary product. The general reaction is as follows:
C5H11N+CH3COCl→C5H10NCOCH3+HCl
In this reaction, piperidine reacts with acetyl chloride to form piperidine, acetate and hydrochloric acid as a byproduct.
Industrial Production Methods
Industrial production of piperidine, acetate often involves the hydrogenation of pyridine in the presence of a catalyst such as molybdenum disulfide. The resulting piperidine is then reacted with acetic anhydride or acetyl chloride to produce piperidine, acetate. This method is efficient and scalable for large-scale production.
Chemical Reactions Analysis
Types of Reactions
Piperidinium acetate undergoes various chemical reactions, including:
Oxidation: this compound can be oxidized to form piperidone, a key intermediate in the synthesis of various pharmaceuticals.
Reduction: Reduction of piperidine, acetate can yield piperidine and acetic acid.
Substitution: this compound can undergo nucleophilic substitution reactions, where the acetate group is replaced by other functional groups.
Common Reagents and Conditions
Oxidation: Common oxidizing agents include potassium permanganate and chromium trioxide.
Reduction: Reducing agents such as lithium aluminum hydride or sodium borohydride are typically used.
Substitution: Nucleophiles such as amines or thiols can be used in substitution reactions.
Major Products Formed
Oxidation: Piperidone
Reduction: Piperidine and acetic acid
Substitution: Various substituted piperidine derivatives
Scientific Research Applications
Piperidinium acetate has numerous applications in scientific research:
Chemistry: It is used as a reagent in organic synthesis and as a building block for the synthesis of complex molecules.
Biology: this compound derivatives are studied for their potential biological activities, including antimicrobial and anticancer properties.
Medicine: It serves as an intermediate in the synthesis of pharmaceuticals, including analgesics, antipsychotics, and antihypertensive agents.
Industry: this compound is used in the production of agrochemicals, dyes, and rubber additives.
Mechanism of Action
The mechanism of action of piperidine, acetate depends on its specific application. In biological systems, piperidine derivatives can interact with various molecular targets, including enzymes and receptors. For example, piperidine-based drugs may inhibit enzyme activity or modulate receptor function, leading to therapeutic effects. The exact pathways involved vary depending on the specific compound and its target.
Comparison with Similar Compounds
Piperidinium acetate can be compared with other similar compounds such as pyridine, pyrrolidine, and piperazine:
Pyridine: A six-membered aromatic heterocycle with one nitrogen atom. Unlike piperidine, pyridine is aromatic and has different reactivity.
Pyrrolidine: A five-membered heterocyclic amine with the molecular formula C4H9N. It is structurally similar to piperidine but has a smaller ring size.
Piperazine: A six-membered heterocyclic compound with two nitrogen atoms at opposite positions. Piperazine has different chemical properties and applications compared to piperidine.
This compound is unique due to its specific reactivity and applications in various fields, making it a valuable compound in both research and industry.
Properties
Molecular Formula |
C7H15NO2 |
---|---|
Molecular Weight |
145.20 g/mol |
IUPAC Name |
acetic acid;piperidine |
InChI |
InChI=1S/C5H11N.C2H4O2/c1-2-4-6-5-3-1;1-2(3)4/h6H,1-5H2;1H3,(H,3,4) |
InChI Key |
RAIYODFGMLZUDF-UHFFFAOYSA-N |
Canonical SMILES |
CC(=O)O.C1CCNCC1 |
Origin of Product |
United States |
Synthesis routes and methods I
Procedure details
Synthesis routes and methods II
Procedure details
Disclaimer and Information on In-Vitro Research Products
Please be aware that all articles and product information presented on BenchChem are intended solely for informational purposes. The products available for purchase on BenchChem are specifically designed for in-vitro studies, which are conducted outside of living organisms. In-vitro studies, derived from the Latin term "in glass," involve experiments performed in controlled laboratory settings using cells or tissues. It is important to note that these products are not categorized as medicines or drugs, and they have not received approval from the FDA for the prevention, treatment, or cure of any medical condition, ailment, or disease. We must emphasize that any form of bodily introduction of these products into humans or animals is strictly prohibited by law. It is essential to adhere to these guidelines to ensure compliance with legal and ethical standards in research and experimentation.