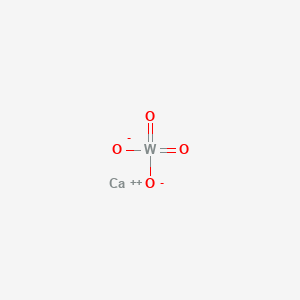
Tungstate calcium (T-4)-lead-doped
- Click on QUICK INQUIRY to receive a quote from our team of experts.
- With the quality product at a COMPETITIVE price, you can focus more on your research.
Overview
Description
Tungstate calcium (T-4)-lead-doped is a chemical compound with the formula CaWO₄. It is a white, crystalline powder that is insoluble in water. This compound is known for its high density and luminescent properties, making it valuable in various industrial and scientific applications. This compound is primarily used as a source of tungsten, a metal with a high melting point and significant industrial importance.
Preparation Methods
Synthetic Routes and Reaction Conditions: Tungstate calcium (T-4)-lead-doped can be synthesized through various methods. One common method involves the reaction between calcium nitrate and sodium tungstate in an aqueous solution. This reaction produces calcium tungstate as a precipitate, which can be filtered and dried. The reaction conditions typically include maintaining a specific pH and temperature to ensure the formation of pure calcium tungstate .
Another method involves the solid-state reaction between calcium carbonate and tungsten trioxide at high temperatures. This method requires precise control of temperature and reaction time to achieve the desired product .
Industrial Production Methods: In industrial settings, calcium tungstate is often produced through large-scale hydrothermal synthesis. This method involves reacting calcium salts with tungstate salts under high pressure and temperature in an autoclave. The hydrothermal method allows for the production of high-purity calcium tungstate with controlled particle size and morphology .
Chemical Reactions Analysis
Types of Reactions: Tungstate calcium (T-4)-lead-doped undergoes various chemical reactions, including oxidation, reduction, and substitution reactions. It can act as a catalyst in oxidation reactions, where it facilitates the conversion of organic substrates to their oxidized forms using hydrogen peroxide as the oxidizing agent .
Common Reagents and Conditions: Common reagents used in reactions involving calcium tungstate include hydrogen peroxide, acids, and bases. The reaction conditions, such as temperature, pH, and concentration of reagents, play a crucial role in determining the outcome of the reactions .
Major Products Formed: The major products formed from reactions involving calcium tungstate depend on the specific reaction conditions and reagents used. For example, in oxidation reactions, the major products are often oxidized organic compounds .
Scientific Research Applications
Tungstate calcium (T-4)-lead-doped has a wide range of scientific research applications. In chemistry, it is used as a catalyst for various organic reactions, including oxidation and reduction reactions . In biology, calcium tungstate nanoparticles are studied for their potential use in drug delivery systems and as imaging agents due to their luminescent properties .
In medicine, calcium tungstate is used in X-ray screens and other imaging devices because of its ability to emit light when exposed to X-rays . In industry, it is used in the production of tungsten metal and its alloys, which are essential for manufacturing hard metals, filaments, and other high-temperature applications .
Mechanism of Action
The mechanism of action of calcium tungstate in catalytic reactions involves the formation of a soluble pertungstate species, which allows for homogeneous reaction conditions. This species facilitates the transfer of oxygen atoms to the substrate, leading to its oxidation . The molecular targets and pathways involved in these reactions depend on the specific substrate and reaction conditions.
Comparison with Similar Compounds
Tungstate calcium (T-4)-lead-doped is often compared with other tungstate compounds, such as sodium tungstate and ammonium tungstate. While all these compounds contain the tungstate anion, their properties and applications differ. For example, sodium tungstate is more soluble in water and is used in different catalytic and industrial applications compared to calcium tungstate .
List of Similar Compounds:- Sodium tungstate (Na₂WO₄)
- Ammonium tungstate ((NH₄)₁₀W₁₂O₄₁)
- Barium tungstate (BaWO₄)
- Strontium tungstate (SrWO₄)
Each of these compounds has unique properties that make them suitable for specific applications, highlighting the versatility and importance of tungstate chemistry.
Properties
CAS No. |
68784-53-2 |
---|---|
Molecular Formula |
CaWO4 CaO4W |
Molecular Weight |
287.92 g/mol |
IUPAC Name |
calcium;dioxido(dioxo)tungsten |
InChI |
InChI=1S/Ca.4O.W/q+2;;;2*-1; |
InChI Key |
FDMFQOCGNBYKPY-UHFFFAOYSA-N |
Canonical SMILES |
[O-][W](=O)(=O)[O-].[Ca+2] |
Origin of Product |
United States |
Synthesis routes and methods
Procedure details
Disclaimer and Information on In-Vitro Research Products
Please be aware that all articles and product information presented on BenchChem are intended solely for informational purposes. The products available for purchase on BenchChem are specifically designed for in-vitro studies, which are conducted outside of living organisms. In-vitro studies, derived from the Latin term "in glass," involve experiments performed in controlled laboratory settings using cells or tissues. It is important to note that these products are not categorized as medicines or drugs, and they have not received approval from the FDA for the prevention, treatment, or cure of any medical condition, ailment, or disease. We must emphasize that any form of bodily introduction of these products into humans or animals is strictly prohibited by law. It is essential to adhere to these guidelines to ensure compliance with legal and ethical standards in research and experimentation.