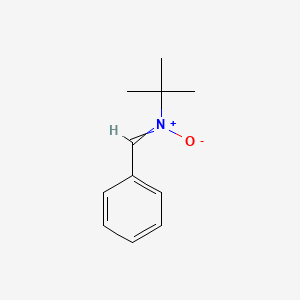
n-tert-butyl-alpha-phenylnitrone
Overview
Description
Preparation Methods
The synthesis of n-tert-butyl-alpha-phenylnitrone typically involves the reaction of tert-butylamine with benzaldehyde under specific conditions . The reaction is carried out in the presence of an oxidizing agent to form the N-oxide derivative. Industrial production methods may involve similar synthetic routes but on a larger scale, with optimized reaction conditions to ensure high yield and purity.
Chemical Reactions Analysis
n-tert-butyl-alpha-phenylnitrone undergoes various chemical reactions, including:
Oxidation: The compound can be further oxidized to form different oxidation products.
Reduction: It can be reduced to its corresponding amine.
Substitution: The phenyl group can undergo electrophilic substitution reactions. Common reagents used in these reactions include oxidizing agents like hydrogen peroxide and reducing agents like sodium borohydride. The major products formed depend on the specific reaction conditions and reagents used.
Scientific Research Applications
n-tert-butyl-alpha-phenylnitrone has several scientific research applications:
Chemistry: It is used as a reagent in organic synthesis and as a precursor for other chemical compounds.
Industry: It is used in the production of various industrial chemicals and materials.
Mechanism of Action
The mechanism of action of n-tert-butyl-alpha-phenylnitrone involves its interaction with molecular targets and pathways in biological systems. It is known to inhibit the activity of certain enzymes and modulate signaling pathways involved in inflammation and oxidative stress . These interactions contribute to its observed biological effects.
Comparison with Similar Compounds
n-tert-butyl-alpha-phenylnitrone can be compared with other similar compounds such as:
N-tert-Butyl-α-phenylnitrone: Shares a similar structure but differs in its specific functional groups.
Phenyl N-tert-butylnitrone: Another closely related compound with similar chemical properties. The uniqueness of this compound lies in its specific N-oxide functional group, which imparts distinct chemical reactivity and biological activity.
Properties
Molecular Formula |
C11H15NO |
---|---|
Molecular Weight |
177.24 g/mol |
IUPAC Name |
N-tert-butyl-1-phenylmethanimine oxide |
InChI |
InChI=1S/C11H15NO/c1-11(2,3)12(13)9-10-7-5-4-6-8-10/h4-9H,1-3H3 |
InChI Key |
IYSYLWYGCWTJSG-UHFFFAOYSA-N |
Canonical SMILES |
CC(C)(C)[N+](=CC1=CC=CC=C1)[O-] |
physical_description |
Solid; [Merck Index] White crystalline needles; [Alfa Aesar MSDS] |
vapor_pressure |
0.00000043 [mmHg] |
Origin of Product |
United States |
Synthesis routes and methods I
Procedure details
Synthesis routes and methods II
Procedure details
Synthesis routes and methods III
Procedure details
Synthesis routes and methods IV
Procedure details
Disclaimer and Information on In-Vitro Research Products
Please be aware that all articles and product information presented on BenchChem are intended solely for informational purposes. The products available for purchase on BenchChem are specifically designed for in-vitro studies, which are conducted outside of living organisms. In-vitro studies, derived from the Latin term "in glass," involve experiments performed in controlled laboratory settings using cells or tissues. It is important to note that these products are not categorized as medicines or drugs, and they have not received approval from the FDA for the prevention, treatment, or cure of any medical condition, ailment, or disease. We must emphasize that any form of bodily introduction of these products into humans or animals is strictly prohibited by law. It is essential to adhere to these guidelines to ensure compliance with legal and ethical standards in research and experimentation.