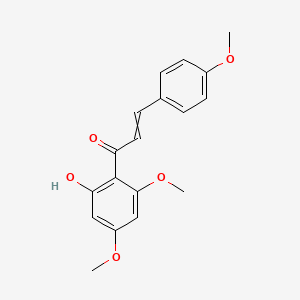
Flavokavain A
Overview
Description
Flavokavain A is a naturally occurring chalcone found in the kava plant (Piper methysticum). It is known for its potential medicinal properties, particularly its ability to induce apoptosis in cancer cells. The compound has been studied for its anti-cancer, anti-inflammatory, and hepatotoxic effects .
Preparation Methods
Synthetic Routes and Reaction Conditions
Flavokavain A can be synthesized through the Claisen-Schmidt condensation reaction. This involves the reaction of 2-hydroxy-4,6-dimethoxyacetophenone with 4-methoxybenzaldehyde in the presence of a base such as sodium hydroxide or potassium hydroxide. The reaction is typically carried out in an ethanol or methanol solvent at room temperature .
Industrial Production Methods
Industrial production of this compound involves the extraction of kava root, followed by purification using chromatographic techniques. Ultra-high-performance liquid chromatography (UHPLC) is commonly used to separate and quantify this compound from other kavalactones and flavokavains present in the kava extract .
Chemical Reactions Analysis
Types of Reactions
Flavokavain A undergoes various chemical reactions, including:
Oxidation: this compound can be oxidized to form quinones, which are reactive intermediates.
Reduction: The compound can be reduced to form dihydrochalcones.
Substitution: this compound can undergo nucleophilic substitution reactions at the methoxy groups.
Common Reagents and Conditions
Oxidation: Common oxidizing agents include potassium permanganate and hydrogen peroxide.
Reduction: Reducing agents such as sodium borohydride or lithium aluminum hydride are used.
Substitution: Nucleophiles such as amines or thiols can be used in substitution reactions.
Major Products
Oxidation: Quinones and other oxidized derivatives.
Reduction: Dihydrochalcones.
Substitution: Various substituted chalcones depending on the nucleophile used.
Scientific Research Applications
Chemistry: Used as a model compound for studying chalcone chemistry and reactivity.
Biology: Investigated for its ability to induce apoptosis in cancer cells, particularly bladder cancer cells.
Medicine: Potential therapeutic agent for cancer treatment due to its pro-apoptotic properties.
Industry: Used in the development of dietary supplements and herbal medicines derived from kava extract.
Mechanism of Action
Flavokavain A exerts its effects primarily through the induction of apoptosis in cancer cells. It activates the Bax protein-dependent and mitochondria-dependent apoptotic pathways. This leads to the loss of mitochondrial membrane potential, release of cytochrome c into the cytosol, and activation of caspases, ultimately resulting in cell death . This compound also down-regulates the expression of anti-apoptotic proteins such as X-linked inhibitor of apoptosis and survivin .
Comparison with Similar Compounds
Flavokavain A is one of three major flavokavains found in kava, the others being Flavokavain B and Flavokavain C. While all three compounds share similar chalcone structures, they exhibit different biological activities:
Flavokavain B: More effective in inducing cell cycle arrest and apoptosis in various cancer cell lines compared to this compound.
Flavokavain C: Exhibits anticancer effects specifically against colon and breast cancer.
This compound is unique in its specific pro-apoptotic activity in bladder cancer cells and its potential hepatotoxicity when combined with paracetamol .
Properties
Molecular Formula |
C18H18O5 |
---|---|
Molecular Weight |
314.3 g/mol |
IUPAC Name |
1-(2-hydroxy-4,6-dimethoxyphenyl)-3-(4-methoxyphenyl)prop-2-en-1-one |
InChI |
InChI=1S/C18H18O5/c1-21-13-7-4-12(5-8-13)6-9-15(19)18-16(20)10-14(22-2)11-17(18)23-3/h4-11,20H,1-3H3 |
InChI Key |
CGIBCVBDFUTMPT-UHFFFAOYSA-N |
SMILES |
COC1=CC=C(C=C1)C=CC(=O)C2=C(C=C(C=C2OC)OC)O |
Canonical SMILES |
COC1=CC=C(C=C1)C=CC(=O)C2=C(C=C(C=C2OC)OC)O |
Origin of Product |
United States |
Synthesis routes and methods
Procedure details
Retrosynthesis Analysis
AI-Powered Synthesis Planning: Our tool employs the Template_relevance Pistachio, Template_relevance Bkms_metabolic, Template_relevance Pistachio_ringbreaker, Template_relevance Reaxys, Template_relevance Reaxys_biocatalysis model, leveraging a vast database of chemical reactions to predict feasible synthetic routes.
One-Step Synthesis Focus: Specifically designed for one-step synthesis, it provides concise and direct routes for your target compounds, streamlining the synthesis process.
Accurate Predictions: Utilizing the extensive PISTACHIO, BKMS_METABOLIC, PISTACHIO_RINGBREAKER, REAXYS, REAXYS_BIOCATALYSIS database, our tool offers high-accuracy predictions, reflecting the latest in chemical research and data.
Strategy Settings
Precursor scoring | Relevance Heuristic |
---|---|
Min. plausibility | 0.01 |
Model | Template_relevance |
Template Set | Pistachio/Bkms_metabolic/Pistachio_ringbreaker/Reaxys/Reaxys_biocatalysis |
Top-N result to add to graph | 6 |
Feasible Synthetic Routes
Disclaimer and Information on In-Vitro Research Products
Please be aware that all articles and product information presented on BenchChem are intended solely for informational purposes. The products available for purchase on BenchChem are specifically designed for in-vitro studies, which are conducted outside of living organisms. In-vitro studies, derived from the Latin term "in glass," involve experiments performed in controlled laboratory settings using cells or tissues. It is important to note that these products are not categorized as medicines or drugs, and they have not received approval from the FDA for the prevention, treatment, or cure of any medical condition, ailment, or disease. We must emphasize that any form of bodily introduction of these products into humans or animals is strictly prohibited by law. It is essential to adhere to these guidelines to ensure compliance with legal and ethical standards in research and experimentation.