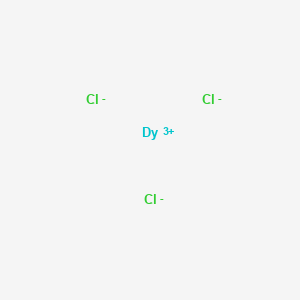
Dysprosium(III) chloride
- Click on QUICK INQUIRY to receive a quote from our team of experts.
- With the quality product at a COMPETITIVE price, you can focus more on your research.
Overview
Description
It appears as a white to yellow solid and is highly hygroscopic, meaning it rapidly absorbs water from the air to form a hexahydrate, DyCl₃·6H₂O . This compound is significant in various scientific and industrial applications due to its unique properties.
Preparation Methods
Synthetic Routes and Reaction Conditions: Dysprosium(III) chloride can be synthesized through several methods:
Ammonium Chloride Route: This method involves reacting dysprosium oxide (Dy₂O₃) with ammonium chloride (NH₄Cl) to produce ammonium pentachlorodysprosate, which is then thermally decomposed to yield this compound. [ 10 \text{NH}_4\text{Cl} + \text{Dy}_2\text{O}_3 \rightarrow 2 (\text{NH}_4)_2[\text{DyCl}_5] + 6 \text{NH}_3 + 3 \text{H}_2\text{O} ] [ (\text{NH}_4)_2[\text{DyCl}_5] \rightarrow 2 \text{NH}_4\text{Cl} + \text{DyCl}_3 ]
Direct Reaction with Hydrochloric Acid: Dysprosium metal or dysprosium carbonate can be reacted with hydrochloric acid to produce this compound. [ 2 \text{Dy} + 6 \text{HCl} \rightarrow 2 \text{DyCl}_3 + 3 \text{H}_2 ] [ \text{Dy}_2(\text{CO}_3)_3 + 6 \text{HCl} \rightarrow 2 \text{DyCl}_3 + 3 \text{CO}_2 + 3 \text{H}_2\text{O} ]
Industrial Production Methods: In industrial settings, this compound is often produced by the ammonium chloride route due to its efficiency and scalability. The compound is typically purified through high-temperature sublimation under vacuum conditions .
Types of Reactions:
Oxidation and Reduction: this compound can undergo reduction to form dysprosium metal when electrolyzed in a molten salt mixture.
Substitution Reactions: It can react with sodium fluoride to form dysprosium(III) fluoride. [ \text{DyCl}_3 + 3 \text{NaF} \rightarrow \text{DyF}_3 + 3 \text{NaCl} ]
Common Reagents and Conditions:
Reducing Agents: Electrolysis in a molten salt mixture (eutectic LiCl-KCl) with a tungsten cathode.
Substitution Reagents: Sodium fluoride (NaF) in aqueous solutions.
Major Products:
Dysprosium Metal: Produced via electrolysis.
Dysprosium(III) Fluoride: Formed through substitution reactions with fluoride salts.
Scientific Research Applications
Dysprosium(III) chloride has a wide range of applications in scientific research:
Chemistry: Used as a starting material for synthesizing other dysprosium compounds.
Biology and Medicine: Investigated for its potential use in magnetic resonance imaging (MRI) contrast agents due to its paramagnetic properties.
Industry: Utilized in the production of magnetostrictive materials and high-performance magnets.
Mechanism of Action
The mechanism of action of dysprosium(III) chloride primarily involves its role as a Lewis acid. It can coordinate with various ligands, forming complexes that are useful in catalysis and materials science . In electrochemical applications, dysprosium(III) ions undergo reduction to form dysprosium metal, which is essential in the production of high-performance magnets .
Comparison with Similar Compounds
- Dysprosium(III) Fluoride (DyF₃)
- Dysprosium(III) Bromide (DyBr₃)
- Dysprosium(III) Iodide (DyI₃)
- Dysprosium(III) Oxide (Dy₂O₃)
Comparison: Dysprosium(III) chloride is unique due to its high solubility in water and its ability to form a hexahydrate. This property makes it particularly useful in aqueous chemistry and for preparing other dysprosium compounds. In contrast, dysprosium(III) fluoride and oxide are less soluble and are typically used in solid-state applications .
Properties
Molecular Formula |
Cl3Dy |
---|---|
Molecular Weight |
268.85 g/mol |
IUPAC Name |
dysprosium(3+);trichloride |
InChI |
InChI=1S/3ClH.Dy/h3*1H;/q;;;+3/p-3 |
InChI Key |
BOXVSFHSLKQLNZ-UHFFFAOYSA-K |
Canonical SMILES |
[Cl-].[Cl-].[Cl-].[Dy+3] |
Origin of Product |
United States |
Disclaimer and Information on In-Vitro Research Products
Please be aware that all articles and product information presented on BenchChem are intended solely for informational purposes. The products available for purchase on BenchChem are specifically designed for in-vitro studies, which are conducted outside of living organisms. In-vitro studies, derived from the Latin term "in glass," involve experiments performed in controlled laboratory settings using cells or tissues. It is important to note that these products are not categorized as medicines or drugs, and they have not received approval from the FDA for the prevention, treatment, or cure of any medical condition, ailment, or disease. We must emphasize that any form of bodily introduction of these products into humans or animals is strictly prohibited by law. It is essential to adhere to these guidelines to ensure compliance with legal and ethical standards in research and experimentation.