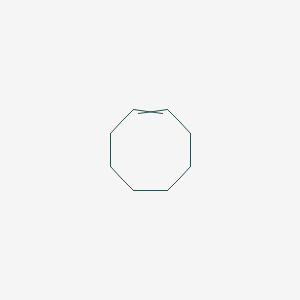
CYCLOOCTENE
Overview
Description
CYCLOOCTENE is a cycloalkene with the molecular formula C₈H₁₄. It consists of a ring of eight carbon atoms, connected by seven single bonds and one double bond. This compound is notable for being the smallest cycloalkene that can exist stably as either the cis or trans stereoisomer, with the cis isomer being the most common . The cyclic structure introduces a point of unsaturation in the molecule, making it an interesting subject for various chemical reactions and applications.
Preparation Methods
CYCLOOCTENE can be synthesized through several methods:
Photoisomerization: This method involves the use of light to convert trans-cyclooctene to cis-cyclooctene.
Ring-Closing Metathesis: In a laboratory setting, the ring-closing metathesis reaction is widely used.
Fractional Distillation and Gas Chromatography: The cis-isomer can be separated from the trans-isomer by fractional distillation through a spinning-band column, followed by preparative gas chromatography.
Chemical Reactions Analysis
CYCLOOCTENE undergoes various types of chemical reactions:
Oxidation: It can be oxidized to form 1,2-epoxycyclooctane using oxidizing agents like hydrogen peroxide in the presence of a tungsten-based catalyst. This reaction involves multiple steps, including epoxidation, hydrolysis, and oxidation.
Addition Reactions: It reacts with halogens (e.g., chlorine or bromine) in the presence of UV light or heat, leading to the formation of halo-cyclooctanes.
Substitution Reactions: cis-Cyclooctene can undergo addition reactions with carbon tetrachloride catalyzed by dichloro tris (triphenylphosphine) ruthenium (II) to yield 1-chloro-4-(trichloromethyl)cyclooctane.
Scientific Research Applications
CYCLOOCTENE has diverse applications in scientific research:
Polymer Science: It is used in the synthesis of polymers and copolymers.
Biocatalysis: It is employed in the biocatalytic epoxidation to produce 1,2-epoxycyclooctane using immobilized Aspergillus niger lipase.
Bioorthogonal Chemistry: The fast reaction between 1,2,4,5-tetrazines and trans-cyclooctenes is frequently used in bioorthogonal ligations, which are valuable in fluorescent imaging, drug delivery, and radiochemistry.
Mechanism of Action
The mechanism of action of CYCLOOCTENE in various reactions involves several steps:
Epoxidation: The double bond in this compound reacts with electrophilic oxidants to form an epoxide.
Hydrolysis and Oxidation: The epoxide can undergo hydrolysis to form a diol, which can further be oxidized to form various products, including dicarboxylic acids.
Comparison with Similar Compounds
CYCLOOCTENE can be compared with other cycloalkenes:
Cyclohexene: A smaller cycloalkene with six carbon atoms. It is less strained compared to this compound due to its smaller ring size.
Cyclodecene: A larger cycloalkene with ten carbon atoms.
Cyclooctane: The saturated counterpart of this compound, lacking the double bond and thus not participating in the same types of reactions.
This compound stands out due to its unique stability as the smallest cycloalkene with cis and trans isomers, making it a valuable compound in both industrial and research settings.
Properties
IUPAC Name |
cyclooctene | |
---|---|---|
Details | Computed by LexiChem 2.6.6 (PubChem release 2019.06.18) | |
Source | PubChem | |
URL | https://pubchem.ncbi.nlm.nih.gov | |
Description | Data deposited in or computed by PubChem | |
InChI |
InChI=1S/C8H14/c1-2-4-6-8-7-5-3-1/h1-2H,3-8H2 | |
Details | Computed by InChI 1.0.5 (PubChem release 2019.06.18) | |
Source | PubChem | |
URL | https://pubchem.ncbi.nlm.nih.gov | |
Description | Data deposited in or computed by PubChem | |
InChI Key |
URYYVOIYTNXXBN-UHFFFAOYSA-N | |
Details | Computed by InChI 1.0.5 (PubChem release 2019.06.18) | |
Source | PubChem | |
URL | https://pubchem.ncbi.nlm.nih.gov | |
Description | Data deposited in or computed by PubChem | |
Canonical SMILES |
C1CCCC=CCC1 | |
Details | Computed by OEChem 2.1.5 (PubChem release 2019.06.18) | |
Source | PubChem | |
URL | https://pubchem.ncbi.nlm.nih.gov | |
Description | Data deposited in or computed by PubChem | |
Molecular Formula |
C8H14 | |
Details | Computed by PubChem 2.1 (PubChem release 2019.06.18) | |
Source | PubChem | |
URL | https://pubchem.ncbi.nlm.nih.gov | |
Description | Data deposited in or computed by PubChem | |
DSSTOX Substance ID |
DTXSID1061310 | |
Record name | Cyclooctene | |
Source | EPA DSSTox | |
URL | https://comptox.epa.gov/dashboard/DTXSID1061310 | |
Description | DSSTox provides a high quality public chemistry resource for supporting improved predictive toxicology. | |
Molecular Weight |
110.20 g/mol | |
Details | Computed by PubChem 2.1 (PubChem release 2021.05.07) | |
Source | PubChem | |
URL | https://pubchem.ncbi.nlm.nih.gov | |
Description | Data deposited in or computed by PubChem | |
CAS No. |
931-88-4 | |
Record name | Cyclooctene | |
Source | EPA DSSTox | |
URL | https://comptox.epa.gov/dashboard/DTXSID1061310 | |
Description | DSSTox provides a high quality public chemistry resource for supporting improved predictive toxicology. | |
Synthesis routes and methods I
Procedure details
Synthesis routes and methods II
Procedure details
Retrosynthesis Analysis
AI-Powered Synthesis Planning: Our tool employs the Template_relevance Pistachio, Template_relevance Bkms_metabolic, Template_relevance Pistachio_ringbreaker, Template_relevance Reaxys, Template_relevance Reaxys_biocatalysis model, leveraging a vast database of chemical reactions to predict feasible synthetic routes.
One-Step Synthesis Focus: Specifically designed for one-step synthesis, it provides concise and direct routes for your target compounds, streamlining the synthesis process.
Accurate Predictions: Utilizing the extensive PISTACHIO, BKMS_METABOLIC, PISTACHIO_RINGBREAKER, REAXYS, REAXYS_BIOCATALYSIS database, our tool offers high-accuracy predictions, reflecting the latest in chemical research and data.
Strategy Settings
Precursor scoring | Relevance Heuristic |
---|---|
Min. plausibility | 0.01 |
Model | Template_relevance |
Template Set | Pistachio/Bkms_metabolic/Pistachio_ringbreaker/Reaxys/Reaxys_biocatalysis |
Top-N result to add to graph | 6 |
Feasible Synthetic Routes
Disclaimer and Information on In-Vitro Research Products
Please be aware that all articles and product information presented on BenchChem are intended solely for informational purposes. The products available for purchase on BenchChem are specifically designed for in-vitro studies, which are conducted outside of living organisms. In-vitro studies, derived from the Latin term "in glass," involve experiments performed in controlled laboratory settings using cells or tissues. It is important to note that these products are not categorized as medicines or drugs, and they have not received approval from the FDA for the prevention, treatment, or cure of any medical condition, ailment, or disease. We must emphasize that any form of bodily introduction of these products into humans or animals is strictly prohibited by law. It is essential to adhere to these guidelines to ensure compliance with legal and ethical standards in research and experimentation.