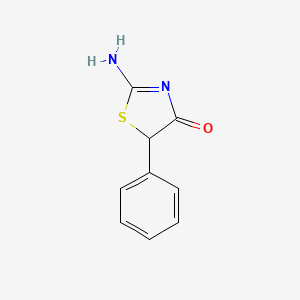
2-amino-5-phenyl-1,3-thiazol-4(5h)-one
- Click on QUICK INQUIRY to receive a quote from our team of experts.
- With the quality product at a COMPETITIVE price, you can focus more on your research.
Overview
Description
2-amino-5-phenyl-1,3-thiazol-4(5h)-one is a heterocyclic compound that contains a thiazole ring, which is a five-membered ring consisting of three carbon atoms, one nitrogen atom, and one sulfur atom The compound is characterized by the presence of an amino group at the second position and a phenyl group at the fifth position of the thiazole ring
Preparation Methods
Synthetic Routes and Reaction Conditions
The synthesis of 2-amino-5-phenyl-1,3-thiazol-4(5h)-one can be achieved through several methods. One common method involves the cyclization of appropriate precursors under specific conditions. For example, the reaction between thiourea and α-bromoacetophenone in the presence of a base such as sodium hydroxide can yield the desired compound. The reaction typically proceeds as follows:
- Dissolve thiourea and α-bromoacetophenone in an appropriate solvent, such as ethanol.
- Add sodium hydroxide to the reaction mixture to initiate the cyclization process.
- Heat the reaction mixture under reflux conditions for several hours.
- After completion of the reaction, cool the mixture and isolate the product by filtration or extraction.
Industrial Production Methods
In an industrial setting, the production of this compound may involve similar synthetic routes but on a larger scale. The use of continuous flow reactors and automated systems can enhance the efficiency and yield of the synthesis process. Additionally, optimization of reaction conditions, such as temperature, pressure, and solvent choice, can further improve the scalability of the production.
Chemical Reactions Analysis
Types of Reactions
2-amino-5-phenyl-1,3-thiazol-4(5h)-one can undergo various chemical reactions, including:
Oxidation: The compound can be oxidized to form corresponding sulfoxides or sulfones.
Reduction: Reduction reactions can lead to the formation of thiazolidines or other reduced derivatives.
Substitution: The amino group and phenyl group can participate in substitution reactions, leading to the formation of various derivatives.
Common Reagents and Conditions
Oxidation: Common oxidizing agents include hydrogen peroxide, m-chloroperbenzoic acid, and potassium permanganate.
Reduction: Reducing agents such as sodium borohydride or lithium aluminum hydride can be used.
Substitution: Electrophilic or nucleophilic reagents, such as alkyl halides or acyl chlorides, can be employed under appropriate conditions.
Major Products
Oxidation: Sulfoxides and sulfones.
Reduction: Thiazolidines and other reduced derivatives.
Substitution: Various substituted thiazole derivatives.
Scientific Research Applications
Chemistry: The compound serves as a building block for the synthesis of more complex molecules and materials.
Biology: It has been investigated for its potential biological activities, including antimicrobial and anticancer properties.
Medicine: The compound is explored for its potential use in drug development, particularly as a scaffold for designing new therapeutic agents.
Industry: It can be used in the development of novel materials with specific properties, such as conductivity or fluorescence.
Mechanism of Action
The mechanism of action of 2-amino-5-phenyl-1,3-thiazol-4(5h)-one depends on its specific application. In medicinal chemistry, the compound may interact with various molecular targets, such as enzymes or receptors, to exert its effects. The presence of the amino and phenyl groups can influence the compound’s binding affinity and specificity for these targets. Additionally, the thiazole ring can participate in various interactions, such as hydrogen bonding and π-π stacking, which contribute to the compound’s overall activity.
Comparison with Similar Compounds
2-amino-5-phenyl-1,3-thiazol-4(5h)-one can be compared with other thiazole derivatives, such as:
2-amino-4-phenylthiazole: Lacks the carbonyl group at the fourth position.
5-phenyl-1,3-thiazol-2-amine: Similar structure but different substitution pattern.
2-amino-5-methyl-1,3-thiazol-4(5h)-one: Contains a methyl group instead of a phenyl group.
Properties
CAS No. |
1762-68-1 |
---|---|
Molecular Formula |
C9H8N2OS |
Molecular Weight |
192.24 g/mol |
IUPAC Name |
2-amino-5-phenyl-1,3-thiazol-4-one |
InChI |
InChI=1S/C9H8N2OS/c10-9-11-8(12)7(13-9)6-4-2-1-3-5-6/h1-5,7H,(H2,10,11,12) |
InChI Key |
YAMZDYXKCLQXKP-UHFFFAOYSA-N |
Canonical SMILES |
C1=CC=C(C=C1)C2C(=O)N=C(S2)N |
Origin of Product |
United States |
Disclaimer and Information on In-Vitro Research Products
Please be aware that all articles and product information presented on BenchChem are intended solely for informational purposes. The products available for purchase on BenchChem are specifically designed for in-vitro studies, which are conducted outside of living organisms. In-vitro studies, derived from the Latin term "in glass," involve experiments performed in controlled laboratory settings using cells or tissues. It is important to note that these products are not categorized as medicines or drugs, and they have not received approval from the FDA for the prevention, treatment, or cure of any medical condition, ailment, or disease. We must emphasize that any form of bodily introduction of these products into humans or animals is strictly prohibited by law. It is essential to adhere to these guidelines to ensure compliance with legal and ethical standards in research and experimentation.