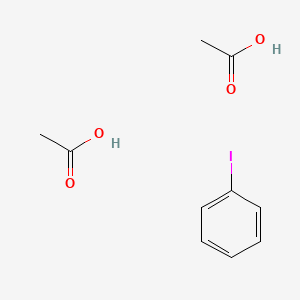
Iodobenzene diacetate
- Click on QUICK INQUIRY to receive a quote from our team of experts.
- With the quality product at a COMPETITIVE price, you can focus more on your research.
Overview
Description
Preparation Methods
Synthetic Routes and Reaction Conditions: Iodobenzene diacetate can be synthesized through several methods. One common method involves the reaction of iodobenzene with a mixture of acetic acid and peracetic acid . Another method uses iodosobenzene and glacial acetic acid . The reaction conditions typically involve moderate temperatures and the use of oxidizing agents such as sodium perborate or potassium peroxydisulfate .
Industrial Production Methods: For industrial production, iodo benzene diacetate is prepared by acylation of iodobenzene using tetrahydrate sodium perborate in the presence of a glacial acetic acid/acetic anhydride mixed solution. The reaction is carried out at temperatures between 30 and 45 degrees Celsius for 4 to 24 hours . The crude product is then purified through recrystallization .
Chemical Reactions Analysis
Types of Reactions: Iodobenzene diacetate undergoes various types of reactions, including oxidation, diacetoxylation, and decarboxylation . It is particularly known for its role as an oxidizing agent in organic synthesis.
Common Reagents and Conditions: Common reagents used in reactions with iodo benzene diacetate include alkenes, aliphatic carboxylic acids, and terminal alkynes . The reactions are typically carried out under mild conditions, often in the presence of catalysts such as copper or rhenium salts .
Major Products: The major products formed from reactions with iodo benzene diacetate include vicinal diacetoxy compounds, allylic acetates, and α-sulfonylamino ketones .
Scientific Research Applications
Iodobenzene diacetate has a wide range of applications in scientific research. It is used in organic synthesis for C-H functionalization, hetero-hetero bond formations, and heterocyclic ring construction . In biology and medicine, it is employed in the synthesis of various pharmaceuticals, including anti-inflammatory drugs and anticancer agents . In the industry, it is used as an oxidizing agent in the production of fine chemicals .
Mechanism of Action
The mechanism of action of iodo benzene diacetate involves the formation of a hypervalent iodine intermediate, which acts as an electrophilic oxidant . This intermediate facilitates the transfer of oxygen atoms to the substrate, leading to the formation of oxidized products . The molecular targets and pathways involved include the activation of alkenes and the formation of carbon-hetero atom bonds .
Comparison with Similar Compounds
Similar Compounds: Similar compounds to iodo benzene diacetate include (bis(trifluoroacetoxy)iodo)benzene and iodosobenzene .
Uniqueness: Iodobenzene diacetate is unique due to its high reactivity and selectivity as an oxidizing agent. It offers mild reaction conditions and a wide substrate scope, making it a versatile reagent in organic synthesis .
Properties
Molecular Formula |
C10H13IO4 |
---|---|
Molecular Weight |
324.11 g/mol |
IUPAC Name |
acetic acid;iodobenzene |
InChI |
InChI=1S/C6H5I.2C2H4O2/c7-6-4-2-1-3-5-6;2*1-2(3)4/h1-5H;2*1H3,(H,3,4) |
InChI Key |
XEFCWBLINXJUIV-UHFFFAOYSA-N |
Canonical SMILES |
CC(=O)O.CC(=O)O.C1=CC=C(C=C1)I |
Origin of Product |
United States |
Synthesis routes and methods
Procedure details
Disclaimer and Information on In-Vitro Research Products
Please be aware that all articles and product information presented on BenchChem are intended solely for informational purposes. The products available for purchase on BenchChem are specifically designed for in-vitro studies, which are conducted outside of living organisms. In-vitro studies, derived from the Latin term "in glass," involve experiments performed in controlled laboratory settings using cells or tissues. It is important to note that these products are not categorized as medicines or drugs, and they have not received approval from the FDA for the prevention, treatment, or cure of any medical condition, ailment, or disease. We must emphasize that any form of bodily introduction of these products into humans or animals is strictly prohibited by law. It is essential to adhere to these guidelines to ensure compliance with legal and ethical standards in research and experimentation.