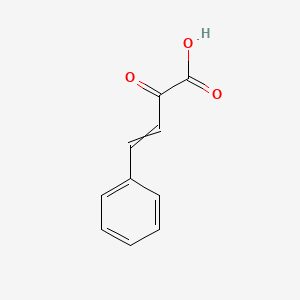
3-Butenoic acid, 2-oxo-4-phenyl-
Overview
Description
3-Butenoic acid, 2-oxo-4-phenyl- (IUPAC name: (3E)-2-oxo-4-phenylbut-3-enoic acid; CAS 17451-19-3) is an α,β-unsaturated ketocarboxylic acid with the molecular formula C₁₀H₈O₃ (average mass: 176.17 g/mol). Its structure features a conjugated double bond (3E configuration), a ketone group at C2, and a phenyl substituent at C4 .
Key physicochemical properties include:
Scientific Research Applications
Pharmaceutical Applications
Intermediates in Drug Synthesis:
3-Butenoic acid, 2-oxo-4-phenyl- serves as an important intermediate in the synthesis of various pharmaceuticals. Specifically, derivatives of this compound are utilized in the production of medicines due to their bioactive properties. For instance, processes for producing 4-phenyl-4-oxo-2-butenoate derivatives highlight their role as key intermediates in drug development .
Anti-inflammatory Properties:
Research indicates that compounds related to 3-butenoic acid, such as 4-phenyl-3-butenoic acid, exhibit anti-inflammatory effects by inhibiting peptidylglycine alpha-amidating monooxygenase. This mechanism has been shown to reduce the growth of certain cancer cell lines, suggesting potential therapeutic applications in oncology .
Agricultural Applications
Pesticide Development:
The derivatives of 3-butenoic acid are also explored for their use in developing pesticides. The ability to synthesize these compounds efficiently and cost-effectively allows for their incorporation into agricultural products aimed at pest control .
Chemical Synthesis
Synthetic Pathways:
The compound is involved in various synthetic pathways that lead to the formation of more complex molecules. For example, a method involving aldol condensation has been documented for producing ethyl 2-oxy-4-phenylbutyrate from 3-butenoic acid derivatives . This highlights its utility in organic synthesis and the development of new chemical entities.
Friedel-Crafts Reactions:
3-Butenoic acid, 2-oxo-4-phenyl-, can be utilized in Friedel-Crafts reactions to generate diverse aromatic compounds, which are crucial in the synthesis of dyes, fragrances, and other industrial chemicals .
Case Study 1: Anti-Tumorigenic Activity
A study on the anti-tumorigenic properties of related compounds demonstrated that they inhibit histone deacetylase enzymes at micromolar concentrations. This suggests that 3-butenoic acid derivatives could be developed further as potential anti-cancer agents .
Case Study 2: Synthesis Efficiency
Research into synthetic methods for producing derivatives of this compound has shown improvements in yield and cost-effectiveness through optimized reaction conditions. For example, using Grignard reactions has led to high yields (over 80%) while minimizing by-products .
Q & A
Basic Research Questions
Q. What are the recommended synthetic routes for 3-butenoic acid, 2-oxo-4-phenyl-, and how can reaction conditions be optimized?
- Methodology : The compound can be synthesized via a two-step protocol: (1) Activation of the carboxylic acid group using dicyclohexylcarbodiimide (DCC) and 4-dimethylaminopyridine (DMAP) in dichloromethane at 20°C for 2 hours. (2) Subsequent coupling with diphenylphosphine oxide under copper(II) oxide catalysis in chloroform at 65°C . Optimize yields by adjusting stoichiometric ratios (e.g., 1:1.65 molar ratio of acid to DCC) and monitoring reaction progress via TLC or HPLC.
Q. How can the purity of 3-butenoic acid, 2-oxo-4-phenyl-, be verified post-synthesis?
- Methodology : Use a combination of chromatographic techniques (e.g., column chromatography with ethyl acetate/hexane gradients) followed by recrystallization from ethanol. Confirm purity via melting point analysis, NMR (¹H/¹³C), and high-resolution mass spectrometry (HRMS). Cross-reference spectral data with PubChem entries for structural validation .
Q. What computational tools are suitable for predicting the physicochemical properties of this compound?
- Methodology : Employ quantum mechanical software (e.g., Gaussian) to calculate dipole moments, logP values, and electrostatic potential surfaces. Use PubChem’s computed properties (e.g., hydrogen bond donors/acceptors, rotatable bonds) to guide experimental design .
Advanced Research Questions
Q. How does the phenyl substituent at the 4-position influence the compound’s reactivity in Michael addition reactions?
- Methodology : Perform kinetic studies using UV-Vis spectroscopy to monitor reaction rates with nucleophiles (e.g., amines or thiols). Compare results with analogous compounds lacking the phenyl group. Computational modeling (DFT) can identify electronic effects (e.g., resonance stabilization of the α,β-unsaturated ketone) .
Q. What experimental strategies can resolve contradictions in reported biological activities (e.g., enzyme inhibition vs. receptor binding)?
- Methodology : Conduct dose-response assays across multiple enzyme systems (e.g., cyclooxygenase-2 for anti-inflammatory activity) and receptor-binding assays (e.g., G-protein-coupled receptors). Use statistical tools (ANOVA) to analyze discrepancies and validate findings with orthogonal techniques like surface plasmon resonance (SPR) .
Q. How can stereochemical outcomes be controlled during asymmetric synthesis of related 4-phenyl derivatives?
- Methodology : Utilize chiral auxiliaries (e.g., Evans oxazolidinones) or enantioselective catalysts (e.g., organocatalysts like proline derivatives). Analyze enantiomeric excess (ee) via chiral HPLC or polarimetry. Reference stereochemical data from structurally similar compounds (e.g., (R)-2-hydroxy-4-phenyl-3-butenoic acid) .
Q. What advanced spectroscopic techniques are critical for characterizing degradation products under oxidative conditions?
- Methodology : Employ LC-MS/MS to identify fragmentation patterns and stable isotope labeling to trace degradation pathways. Use X-ray crystallography (as in chromene-carboxylic acid derivatives) to resolve ambiguous structures .
Q. Key Considerations
- Contradictions : Discrepancies in hydrogen bond counts (e.g., 2 donors in vs. 3 in other analogs) may arise from tautomerism or computational settings. Validate experimentally .
- Safety : Follow OSHA guidelines for handling α,β-unsaturated ketones (potential sensitizers). Use fume hoods and PPE during synthesis .
Comparison with Similar Compounds
Comparison with Structurally Similar Compounds
4-Phenyl-4-oxo-2-butenoic Acid Derivatives
- Structure : Features a ketone at C4 and a double bond at C2-C3 (vs. C3-C4 in the main compound).
- Biological Activity : Acts as a kynurenine-3-hydroxylase inhibitor , making it relevant in treating neurodegenerative diseases by modulating tryptophan metabolism .
- Key Difference: The shifted oxo group (C4 vs.
4-Phenyl-3-butenoic Acid (PBA)
- Structure : Lacks the C2 oxo group but retains the phenyl group at C4 and the C3-C4 double bond.
- Biological Activity: Inhibits peptidylglycine α-monooxygenase (PAM), reducing neuropeptide amidation and exhibiting anti-inflammatory and analgesic effects in rodent models .
4-Oxo-4-phenylbutyric Acid (3-Benzoylpropionic Acid)
- Applications : Used in organic synthesis and as a pharmaceutical intermediate.
- Key Difference : Saturation eliminates conjugation, reducing UV activity and altering metabolic stability.
4-(4-Bromophenyl)-4-oxo-but-2-enoic Acid
- Structure : Substituted with a bromine atom on the phenyl ring and a double bond at C2-C3.
- Synthetic Utility : Serves as a precursor for heterocyclic compounds (e.g., benzoxazoles and thiadiazoles) .
- Key Difference : Bromine enhances electron-withdrawing effects, increasing acidity of the carboxylic acid group (pKa ~3.5 vs. ~4.2 for the main compound).
Ethyl 3-Oxo-4-phenylbutanoate
- Structure : Ester derivative of 4-phenyl-3-oxobutyric acid (C12H14O3; molecular weight: 206.24 g/mol) .
- Applications : Improved lipophilicity makes it suitable for drug delivery systems.
- Key Difference : Esterification reduces hydrogen-bonding capacity, altering bioavailability compared to the carboxylic acid form.
Data Tables
Table 1: Structural and Physicochemical Comparison
Discussion of Key Differences
- Reactivity: The conjugated system in 2-oxo-4-phenyl-3-butenoic acid enhances its susceptibility to nucleophilic attack at the β-carbon, unlike saturated analogs like 4-oxo-4-phenylbutyric acid .
- Bioactivity : The C2 oxo group in the main compound enables hydrogen bonding with enzyme active sites, critical for its inhibitory effects compared to PBA, which lacks this moiety .
- Synthetic Utility: Brominated derivatives (e.g., 4-(4-bromophenyl)-4-oxo-but-2-enoic acid) exhibit enhanced electrophilicity, facilitating cross-coupling reactions .
Preparation Methods
Synthesis via Aldol Condensation of Benzaldehyde and Pyruvate Derivatives
Base-Catalyzed Condensation
The most widely documented method involves the condensation of benzaldehyde with sodium pyruvate in an alkaline aqueous medium. This reaction proceeds via an aldol-like mechanism, forming the α,β-unsaturated γ-keto acid backbone. In a representative procedure, sodium pyruvate (0.55 mol) is dissolved in cold 1N sodium hydroxide (550 mL) at 0°C, followed by the addition of benzaldehyde (0.55 mol) . The mixture is stirred for 24 hours, after which the product precipitates as a sodium salt. Acidification with hydrochloric acid liberates the free acid, which is extracted with diethyl ether and purified by recrystallization .
This method achieves a crude yield of 91%, though purity depends on stringent pH control during acidification. Side products, such as phenyl acetic acid, may form via over-reduction or decarboxylation but are minimized at low temperatures (0–5°C) .
Esterification and Selective Hydrogenation
To enhance stability and facilitate purification, the unsaturated γ-keto acid is often esterified. Treatment with methyl chloroformate in the presence of triethylamine converts the acid to its methyl ester . Subsequent hydrogenation over palladium hydride (Pd-H) selectively reduces the α,β-unsaturated bond without affecting the ketone group, yielding methyl 2-oxo-4-phenylbutanoate (85% yield) . The selectivity of Pd-H contrasts with palladium on carbon (Pd/C), which reduces both the double bond and ketone .
Carbonylation of Benzyl Chloride with Cobalt Catalysis
Reaction Mechanism and Solvent System
An alternative industrial route involves the carbonylation of benzyl chloride under cobalt carbonyl catalysis. The reaction employs a binary solvent system (water and methyl isobutyl ketone) with calcium hydroxide as the base . Cobalt carbonyl (Co₂(CO)₈) facilitates the insertion of carbon monoxide into the benzyl chloride C–Cl bond, generating a benzyl cobalt intermediate that undergoes carboxylation to form the γ-keto acid .
Key advantages of this method include:
-
Catalyst recyclability : The cobalt catalyst remains in the organic phase post-reaction, enabling reuse without regeneration .
-
Mild conditions : Reactions proceed efficiently at 25–50°C under atmospheric pressure, avoiding energy-intensive setups .
Workup and Byproduct Management
Post-reaction, the mixture is filtered to isolate the calcium salt of 3-butenoic acid, 2-oxo-4-phenyl-. Acidification with dilute HCl releases the free acid, which is extracted into diethyl ether . The aqueous phase contains phenyl acetic acid (a byproduct), which is separately recovered by acidification and extraction . This method achieves a 91% yield for the intermediate calcium salt, though the free acid yield drops to 75–80% due to losses during extraction .
Isotopic Synthesis via ¹³C-Labeled Precursors
¹³C-Incorporation for Tracer Studies
A specialized route for synthesizing ¹³C-labeled 3-butenoic acid, 2-oxo-4-phenyl- involves U-¹³C-succinic acid as a starting material . Reaction with thionyl chloride (SOCl₂) generates the acyl chloride, which undergoes Friedel-Crafts acylation with benzene to form U-¹³C-4-oxo-4-phenylbutanoic acid . Wolff-Kishner reduction with hydrazine and potassium hydroxide in diethylene glycol removes the ketone group, yielding U-¹³C-4-phenylbutanoic acid . While this method is critical for isotopic labeling, its complexity limits industrial application.
Comparative Analysis of Preparation Methods
Yield and Scalability
The aldol method offers superior yields and simplicity but requires precise temperature control. Carbonylation excels in catalyst reuse but involves hazardous CO gas. Isotopic synthesis is niche, catering to tracer studies .
Industrial Feasibility
Carbonylation is favored for large-scale production due to solvent recovery and catalyst recyclability . In contrast, aldol condensation is preferred for laboratory-scale synthesis where high purity is paramount .
Challenges and Optimization Strategies
Byproduct Suppression
Phenyl acetic acid formation remains a challenge in both aldol and carbonylation routes. Strategies include:
-
Low-temperature operation : Minimizes decarboxylation in aldol reactions .
-
Phase separation : Binary solvent systems in carbonylation isolate byproducts into aqueous phases .
Catalyst Degradation
Cobalt carbonyl decomposes under acidic conditions, necessitating pH-neutral workup steps . Palladium hydride catalysts require inert atmospheres to prevent oxidation during hydrogenation .
Properties
Molecular Formula |
C10H8O3 |
---|---|
Molecular Weight |
176.17 g/mol |
IUPAC Name |
2-oxo-4-phenylbut-3-enoic acid |
InChI |
InChI=1S/C10H8O3/c11-9(10(12)13)7-6-8-4-2-1-3-5-8/h1-7H,(H,12,13) |
InChI Key |
YQOUMBVFEWZLME-UHFFFAOYSA-N |
Canonical SMILES |
C1=CC=C(C=C1)C=CC(=O)C(=O)O |
Origin of Product |
United States |
Synthesis routes and methods I
Procedure details
Synthesis routes and methods II
Procedure details
Disclaimer and Information on In-Vitro Research Products
Please be aware that all articles and product information presented on BenchChem are intended solely for informational purposes. The products available for purchase on BenchChem are specifically designed for in-vitro studies, which are conducted outside of living organisms. In-vitro studies, derived from the Latin term "in glass," involve experiments performed in controlled laboratory settings using cells or tissues. It is important to note that these products are not categorized as medicines or drugs, and they have not received approval from the FDA for the prevention, treatment, or cure of any medical condition, ailment, or disease. We must emphasize that any form of bodily introduction of these products into humans or animals is strictly prohibited by law. It is essential to adhere to these guidelines to ensure compliance with legal and ethical standards in research and experimentation.