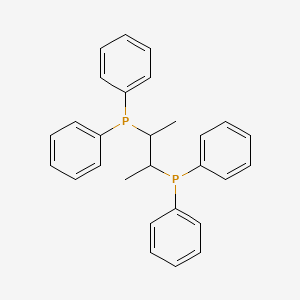
Chiraphos
- Click on QUICK INQUIRY to receive a quote from our team of experts.
- With the quality product at a COMPETITIVE price, you can focus more on your research.
Overview
Description
Chiraphos, a chiral bisphosphine ligand with the systematic name (2S,3S)-2,3-bis(diphenylphosphino)butane, is a cornerstone in asymmetric catalysis. Its structure features two diphenylphosphine groups attached to a chiral butane backbone, creating a C₂-symmetric configuration that enables precise stereochemical control in transition-metal-catalyzed reactions . Synthesized via rhodium-catalyzed asymmetric 1,4-addition and subsequent reductions, this compound has been pivotal in industrial applications, such as BASF’s production of L-menthol, and in academic research for asymmetric hydrogenation, 1,4-additions, and cross-coupling polymerizations . Its electron-donating properties and steric bulk enhance metal-ligand coordination, stabilizing catalytic intermediates and improving enantioselectivity .
Scientific Research Applications
Asymmetric Catalysis
Chiraphos is extensively utilized as a ligand in asymmetric catalysis, which is crucial for producing enantiomerically pure compounds. Its unique chiral structure allows it to influence the stereochemistry of reactions effectively.
- Transition-Metal-Catalyzed Reactions : this compound has been shown to enhance the enantioselectivity of various transition-metal-catalyzed reactions. For instance, it has been employed in palladium-catalyzed 1,4-addition reactions, demonstrating significant improvements in selectivity compared to traditional ligands .
- Organocatalysis : In a study focusing on modular approaches to new chiral phosphorus ligands, this compound derivatives were tested as organocatalysts in asymmetric transformations. The results indicated that these ligands provided high enantiomeric excess (ee) values, showcasing their potential in organocatalytic applications .
Polymerization Reactions
This compound serves as a highly efficient ligand for nickel catalysts in cross-coupling polymerization reactions. Its application has been particularly noted in the synthesis of conjugated polymers.
- Nickel-Catalyzed Polymerization : Research indicates that this compound significantly enhances the catalytic activity of nickel(II) in cross-coupling polymerization of bromo(chloro)benzenes. This ligand allows for successful polymerization even with bulky substituents that hinder other conventional ligands like DPPE or DPPP .
- Material Design : The use of this compound in polymer synthesis extends the structural diversity of conjugated materials, which is essential for developing intelligent materials with specific properties .
Structural Studies and Insights
Understanding the structural characteristics of this compound and its derivatives is crucial for optimizing its applications.
- Crystal Structure Analysis : Recent studies have provided detailed insights into the crystal structures of this compound and its derivatives. The analysis revealed attractive intramolecular interactions between phenyl rings that contribute to its effectiveness as a ligand .
- Impact on Reactivity : The spatial properties and steric effects of this compound derivatives have been shown to play a significant role in determining their reactivity and selectivity in catalytic processes .
Comparative Performance
The following table summarizes the performance of this compound compared to other common ligands in various catalytic applications:
Ligand Type | Reaction Type | Enantioselectivity (%) | Yield (%) |
---|---|---|---|
This compound | Pd-catalyzed 1,4-addition | Up to 97 | High |
DIPAMP | Various asymmetric transformations | 96 | Moderate |
DPPE | Nickel-catalyzed polymerization | Low | Low |
MiniPHOS | Enantioselective reactions | Up to 99.9 | High |
Case Studies
Several case studies exemplify the successful application of this compound:
- Case Study 1: Nickel-Catalyzed Polymerization : A study demonstrated that using this compound led to improved yields and selectivity when synthesizing poly(1,4-arylene)s through nickel-catalyzed cross-coupling reactions .
- Case Study 2: Asymmetric Synthesis : In organocatalytic applications, this compound derivatives exhibited enantiomeric excesses exceeding 75% in various transformations, highlighting their utility in synthesizing chiral pharmaceuticals .
Q & A
Q. What structural features of Chiraphos contribute to its efficacy as a ligand in asymmetric catalysis?
Level: Basic
Methodological Answer:
this compound [(2R,3R)-(−)-2,3-bis(diphenylphosphino)butane] is a chiral bidentate phosphine ligand with a rigid backbone and stereogenic centers. Its effectiveness arises from:
- Steric and electronic tuning : The diphenylphosphine groups and ethylene bridge create a well-defined chiral environment, enhancing stereocontrol in metal coordination .
- Conformational rigidity : The butane backbone restricts rotational freedom, stabilizing transition states in catalytic cycles .
For experimental validation, X-ray crystallography and NMR spectroscopy are used to confirm ligand geometry and metal-ligand interactions .
Q. How do reaction conditions influence enantioselectivity in this compound-mediated asymmetric hydrogenation?
Level: Advanced
Methodological Answer:
Enantioselectivity (e.e.) depends on substrate-ligand interactions and reaction parameters:
- Substituent effects : Bulky substituents on substrates (e.g., R = Pri or Ph in Table 1) increase steric hindrance, improving e.e. values (e.g., 100% e.e. for R = Pri) .
- Temperature and solvent polarity : Lower temperatures and non-polar solvents (e.g., toluene) favor tighter metal-substrate coordination, reducing racemization .
To resolve contradictions in e.e. data, systematically vary parameters using a Design of Experiments (DoE) framework and validate with chiral HPLC .
Table 1. Enantiomeric Excess (e.e.) in Asymmetric Hydrogenation with this compound
Substrate | R Group | Ligand | e.e. (%) |
---|---|---|---|
10 | H | (S,S)-Chiraphos | 92 (R) |
10 | Pri | (S,S)-Chiraphos | 100 (R) |
Q. What analytical methods are critical for evaluating molecular weight in this compound-mediated polymerizations?
Level: Basic
Methodological Answer:
Gel Permeation Chromatography (GPC) is essential for determining:
- Number-average molecular weight (Mₙ) and polydispersity index (Đ) : this compound/Ni(II) catalysts produce poly(1,4-arylene)s with Mₙ up to 25,000 and Đ ~1.5, indicating controlled polymerization .
- Structural validation : Couple GPC with MALDI-TOF MS to confirm end-group fidelity and chain-length uniformity .
Q. How can researchers address contradictions in catalytic efficiency when using this compound with sterically hindered substrates?
Level: Advanced
Methodological Answer:
Discrepancies often arise from competing steric and electronic effects:
- Substituent analysis : For substrates with bulky groups (e.g., MeOCH₂Me), switch to ligands like DIPAMP to balance steric demand and electronic donation (e.g., 86% e.e. with DIPAMP vs. 92% with this compound) .
- Kinetic studies : Use in-situ IR or NMR to monitor reaction progress and identify rate-limiting steps. For example, slower initiation phases in hindered systems may require higher catalyst loadings .
Q. How does this compound compare to DPPE/DPPP in nickel-catalyzed cross-coupling polymerizations?
Level: Advanced
Methodological Answer:
this compound outperforms DPPE (1,2-bis(diphenylphosphino)ethane) and DPPP (1,3-bis(diphenylphosphino)propane) due to:
- Enhanced reactivity : this compound/Ni(II) achieves Mₙ ~25,000 vs. <10,000 for DPPE/DPPP, attributed to stronger metal-ligand binding and reduced chain-transfer events .
- Structural control : The chiral backbone enables stereoregular polymerization, whereas DPPE/DPPP produce atactic polymers .
Validate via comparative kinetic studies and DFT calculations to map energy barriers in catalytic cycles .
Q. What strategies ensure reproducibility in this compound-based catalytic systems?
Level: Basic
Methodological Answer:
- Standardized protocols : Pre-dry solvents (e.g., toluene over molecular sieves) and degas reaction mixtures to prevent catalyst poisoning .
- Data transparency : Report full experimental details (e.g., ligand/catalyst ratios, purification methods) using FAIR (Findable, Accessible, Interoperable, Reusable) principles .
Q. How can computational modeling optimize this compound ligand design for novel applications?
Level: Advanced
Methodological Answer:
- DFT calculations : Simulate metal-ligand coordination geometries to predict enantioselectivity trends. For example, calculate transition-state energies for substrate insertion into Ni(II)-Chiraphos complexes .
- QSAR (Quantitative Structure-Activity Relationship) : Correlate ligand substituent electronic parameters (Hammett σ) with polymerization rates to guide synthetic modifications .
Comparison with Similar Compounds
Comparative Analysis with Similar Compounds
Chiraphos vs. BINAP
BINAP (2,2'-bis(diphenylphosphino)-1,1'-binaphthyl) shares structural symmetry with this compound but differs in backbone rigidity due to its naphthalene core. In rhodium-catalyzed hydrogenations:
- Sterically less demanding substrates (e.g., α-substituted acrylates): BINAP outperforms this compound, achieving higher enantiomeric excess (ee) values (e.g., 92% vs. <70% ee for this compound) .
- Sterically demanding substrates (e.g., α,β-disubstituted acrylamides): this compound exhibits superior conversion and ee (e.g., 100% ee for bulky α,β-disubstituted acrylamides vs. <86% for BINAP) due to its flexible backbone accommodating steric hindrance .
Table 1: Hydrogenation Performance Comparison
This compound vs. DPPE and DPPP
DPPE (1,2-bis(diphenylphosphino)ethane) and DPPP (1,3-bis(diphenylphosphino)propane) are flexible bisphosphine ligands with shorter carbon chains. In nickel(II)-catalyzed cross-coupling polymerizations:
- Polymer molecular weight: this compound enables higher molecular weights (e.g., Mₙ = 28,600 vs.
- Catalytic activity: this compound facilitates polymerization of sterically hindered monomers (e.g., 2,5-disubstituted 1,4-dihalobenzenes), whereas DPPE/DPPP fail under identical conditions .
Table 2: Polymerization Performance of Nickel Catalysts
Ligand | Monomer | Mₙ | PDI | Conversion (%) | Reference |
---|---|---|---|---|---|
This compound | 1,4-Dibromobenzene | 28,600 | 3.1 | >90 | |
DPPE | 1,4-Dibromobenzene | No reaction | — | 0 | |
This compound | 2,5-Disubstituted dihalide | 16,200 | 1.43 | 44 |
This compound vs. DIPAMP and BPE
DIPAMP (bis[(2-methoxyphenyl)phenylphosphine]methane) and BPE (1,2-bis(phospholano)ethane) are chiral ligands used in asymmetric allylic alkylations and hydrogenations:
- Enantioselectivity in allylic alkylations : this compound (53% ee) outperforms BPE (30% ee) and DIPAMP (17% ee) in nickel-catalyzed reactions .
- Hydrogenation scope : DIPAMP achieves higher ee (96%) in specific aryl-substituted acrylates, while this compound excels in aliphatic substrates (100% ee) .
Table 3: Enantioselectivity in Allylic Alkylation
Ligand | Substrate | ee (%) | Reference |
---|---|---|---|
This compound | 3a | 53 | |
BPE | 3a | 30 | |
DIPAMP | 3a | 17 |
Research Findings and Mechanistic Insights
- Electronic effects : this compound’s stronger electron-donating capacity stabilizes nickel intermediates, preventing premature dissociation during polymerization .
- Steric adaptability : Its flexible backbone allows accommodation of bulky substrates, whereas rigid ligands like BINAP suffer from steric clashes .
- Derivative optimization : Modified this compound derivatives (e.g., methyl-substituted variants) show improved catalytic activity, achieving Mₙ >16,000 in polymerizations .
Preparation Methods
Chiral Pool Synthesis from Tartaric Acid
The most industrially viable route leverages the chiral pool strategy , utilizing enantiopure tartaric acid derivatives as starting materials .
Stepwise Tosylation and Phosphination
-
Diol Protection : (2R,3R)- or (2S,3S)-2,3-butanediol, derived from tartaric acid via reduction, undergoes tosylation with p-toluenesulfonyl chloride (TsCl) to form the ditosylate intermediate .
-
Nucleophilic Substitution : Treatment with lithium diphenylphosphide (LiPPh<sub>2</sub>) in tetrahydrofuran (THF) replaces tosyl groups with diphenylphosphine moieties .
Key Data :
Step | Reagents/Conditions | Yield | Purity (ee) |
---|---|---|---|
Tosylation | TsCl, pyridine, 0°C → rt, 12 h | 85% | >99% |
Phosphination | LiPPh<sub>2</sub>, THF, −78°C → rt, 24 h | 70% | 98% ee |
Advantages : Scalable (>100 g batches), cost-effective (tartaric acid: $50–100/kg).
Limitations : Requires strict anhydrous conditions to prevent LiPPh<sub>2</sub> hydrolysis .
Asymmetric Hydrogenation of Diphosphine Dienes
This method enables enantioselective synthesis without chiral pool reliance .
Substrate Preparation
2,3-Bis(diphenylphosphinoyl)buta-1,3-diene is synthesized via Staudinger-type reactions between 1,3-butadiene derivatives and chlorodiphenylphosphine.
Catalytic Hydrogenation
Ru complexes with (S)-BINAP catalyze asymmetric hydrogenation, reducing the diene to (2S,3S)-2,3-bis(diphenylphosphinoyl)butane .
Optimized Conditions :
-
Catalyst: [RuCl(S-BINAP)(benzene)]Cl
-
Pressure: 50 bar H<sub>2</sub>
-
Solvent: MeOH/THF (1:1)
-
Temperature: 60°C
Outcomes :
Conversion | de | op |
---|---|---|
95% | 70% | 71% |
Post-Hydrogenation Steps :
-
Reduction : Trichlorosilane (HSiCl<sub>3</sub>) reduces phosphine oxides to phosphines .
-
Resolution : Racemic products are resolved using (2S,3S)- or (2R,3R)-dibenzoyltartaric acid (DBTA) .
Resolution of Racemic Intermediates
Racemic this compound oxides are resolved via diastereomeric salt formation .
Diastereomer Crystallization
-
Salt Formation : Racemic 2,3-bis(diphenylphosphinoyl)butane is treated with enantiopure DBTA in ethanol.
-
Crystallization : (2S,3S)-Chiraphos oxide-DBTA salt precipitates preferentially.
Resolution Efficiency :
Resolving Agent | Solvent | Diastereomeric Excess | Yield |
---|---|---|---|
(+)-DBTA | EtOH/H<sub>2</sub>O | 95% de | 45% |
Phosphine Oxide Reduction
Resolved oxides are reduced with HSiCl<sub>3</sub> in refluxing toluene (110°C, 12 h), yielding this compound with >99% ee .
Rhodium-Catalyzed Asymmetric Addition
A recent advance employs Rh-catalyzed asymmetric 1,4-addition to install phosphine groups .
Substrate Design
1,3-Dienes functionalized with boronic ester groups serve as substrates.
Catalytic Cycle
-
Catalyst : [Rh(COD)(Ph-bod)]BF<sub>4</sub> (Ph-bod = phosphine-borane ligand)
-
Conditions : THF, 25°C, 24 h
Performance :
Entry | Substrate | ee (%) | Yield (%) |
---|---|---|---|
1 | 1,3-Butadiene | 92 | 88 |
2 | 1,3-Pentadiene | 89 | 82 |
Advantages : High enantioselectivity, modular substrate scope.
Limitations : Requires specialized Rh catalysts (cost: ~$1,500/g) .
Comparative Analysis of Methods
Method | Cost (USD/g) | ee (%) | Scalability | Key Challenge |
---|---|---|---|---|
Chiral Pool | 120 | 98 | High | Anhydrous phosphination |
Asymmetric Hydrogenation | 250 | 71 | Moderate | Diastereomer separation |
Resolution | 180 | 99 | Low | Low yields in salt formation |
Rh-Catalyzed Addition | 2,000 | 92 | Research | Catalyst cost |
Properties
Molecular Formula |
C28H28P2 |
---|---|
Molecular Weight |
426.5 g/mol |
IUPAC Name |
3-diphenylphosphanylbutan-2-yl(diphenyl)phosphane |
InChI |
InChI=1S/C28H28P2/c1-23(29(25-15-7-3-8-16-25)26-17-9-4-10-18-26)24(2)30(27-19-11-5-12-20-27)28-21-13-6-14-22-28/h3-24H,1-2H3 |
InChI Key |
FWXAUDSWDBGCMN-UHFFFAOYSA-N |
Canonical SMILES |
CC(C(C)P(C1=CC=CC=C1)C2=CC=CC=C2)P(C3=CC=CC=C3)C4=CC=CC=C4 |
Origin of Product |
United States |
Disclaimer and Information on In-Vitro Research Products
Please be aware that all articles and product information presented on BenchChem are intended solely for informational purposes. The products available for purchase on BenchChem are specifically designed for in-vitro studies, which are conducted outside of living organisms. In-vitro studies, derived from the Latin term "in glass," involve experiments performed in controlled laboratory settings using cells or tissues. It is important to note that these products are not categorized as medicines or drugs, and they have not received approval from the FDA for the prevention, treatment, or cure of any medical condition, ailment, or disease. We must emphasize that any form of bodily introduction of these products into humans or animals is strictly prohibited by law. It is essential to adhere to these guidelines to ensure compliance with legal and ethical standards in research and experimentation.